You are here: Home > Section on Cellular Differentiation
Molecular Genetics of Heritable Human Disorders
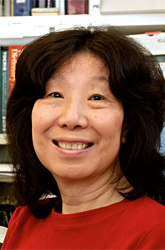
- Janice Y. Chou, PhD, Head, Section on Cellular Differentiation
- Hyun-Sik Jun, PhD, Staff Scientist
- Jun Ho Cho, PhD, Visiting Fellow
- Goo Young Kim, PhD, Visiting Fellow
- Yong Jun Lee, PhD, Visiting Fellow
- Young Mok Lee, PhD, Visiting Fellow
- Brian C. Mansfield, PhD, Guest Researcher
- Chi-Jiunn Pann, BS, Senior Research Assistant
We conduct research to delineate the pathophysiology of glycogen storage disease type I (GSD-I) and glucose-6-phosphatase-β (G6Pase-β or G6PC3) deficiency and to develop novel therapies for these disorders. GSD-I consists of two subtypes, GSD-Ia, deficient in G6Pase-α (or G6PC), and GSD-Ib, deficient in the glucose-6-phosphate (G6P) transporter (G6PT). A third disease, G6Pase-β deficiency, also known as severe congenital neutropenia syndrome type 4 (SCN4) is also grouped with GSD-I. G6Pase-α and G6Pase-β are endoplasmic reticulum (ER)–bound G6P hydrolases, with active sites lying inside the lumen, which depend upon G6PT to translocate G6P from the cytoplasm into the ER lumen. The G6PT/G6Pase-α complex maintains interprandial glucose homeostasis, while the G6PT/G6Pase-β complex maintains energy homeostasis and functionality of neutrophils and macrophages. GSD-Ia and GSD-Ib patients manifest a common metabolic phenotype of impaired glucose homeostasis not shared by G6Pase-β deficiency. GSD-Ib and G6Pase-β-deficient patients manifest a common myeloid phenotype of neutropenia and myeloid dysfunction not shared by GSD-Ia. Neutrophils express the G6PT/G6Pase-β complex, and inactivation of G6PT or G6Pase-β leads to the enhanced neutrophil apoptosis that underlies neutropenia in GSD-Ib and G6Pase-β deficiency. We also showed that G6Pase-β is essential for energy homeostasis in neutrophils and macrophages. A G6Pase-β deficiency prevents recycling of ER glucose to the cytoplasm, leading to neutrophil/macrophage dysfunction. There is no cure for GSD-Ia, GSD-Ib, and G6Pase-β deficiency. Animal models of the three disorders are available and are being exploited to both delineate the disease more precisely and develop new treatment approaches, including gene therapy.
G6Pase-β is essential for energy homeostasis in macrophages and the pregnant uterus; deficiency leads to reduced fertility in G6pc3-/- mothers.
In addition to neutropenia and neutrophil dysfunction, G6Pase-β–deficient patients also exhibit a distinct phenotype of increased visibility of superficial veins, congenital heart defects, and urogenital malformations, suggesting that G6Pase-β deficiency underlies a broader cell dysfunction. The ubiquitous pattern of G6Pase-β expression suggests that G6Pase-β plays a critical role in non-gluconeogenic tissues, where there may be increased demands for glucose. Macrophages play key roles in innate immunity, inflammation, and tissue remodeling. During pregnancy, macrophages also influence the homeostasis of the developing placenta and are important in preventing premature fetal rejection. Macrophages have increased demand for glucose, and pregnancy is a condition with increased demand for glucose. We hypothesized that the cycling pathway for G6P metabolism (Figure 1) that regulates cellular energy homeostasis also occurs in macrophages and pregnancy uterus and that deficiency in G6Pase-β results in macrophage dysfunction and pregnancy-associated complications. This was proven to be the case. We showed that murine G6pc3−/− macrophages exhibit impairments in their respiratory burst, chemotaxis, calcium flux, and phagocytic activities. Consistent with a G6P metabolism deficiency, G6pc3−/− macrophages also exhibit lower glucose uptake, and lower levels of G6P, lactate, and ATP than wild-type macrophages. Furthermore, the expression and activation of NADPH oxidase activity is down-regulated. We further showed that, during pregnancy, the absence of G6Pase-β activity impaired energy homeostasis in the uterus, resulting in reduced production of monocyte chemoattractant protein 1 and macrophage colony-stimulating factor, cytokines that recruit macrophages to a site of injury or inflammation. We found evidence supporting this finding, namely that G6pc3−/− macrophages exhibit repressed trafficking in vivo both during an inflammatory response and in pregnancy. The dysfunctional macrophages and impaired uterine energy homeostasis correlate with reduced fertility in G6pc3−/− mothers.
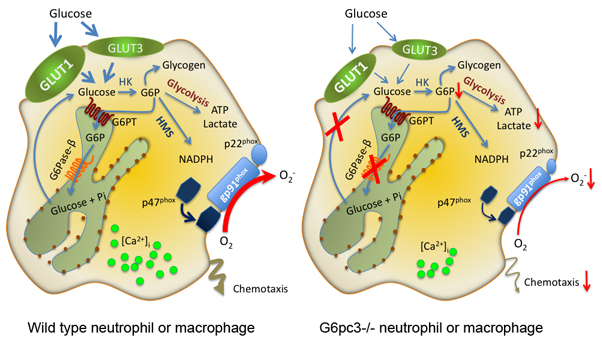
Figure 1. Schematic representation elucidating the cycling pathway for G6P metabolism in neutrophils/macrophages
G6P participates in four major pathways: glycolysis, hexose monophosphate shunt (HMS), glycogen synthesis, or ER cycling. In cycling, G6P enters the ER via G6PT, where G6P can accumulate until it is hydrolyzed to glucose by G6Pase-β and transported back into the cytoplasm. By limiting cytoplasmic glucose/G6P availability, cycling regulates the other three cytoplasmic pathways for G6P metabolism. Disruption of the cycling in G6Pase-β–deficient neutrophils/macrophages results in impaired energy homeostasis and functionality.
Gene therapy mediated by rAAV prevents hepatocellular adenoma and corrects metabolic abnormalities in GSD-Ia mice.
GSD-Ia patients deficient in G6Pase-α (G6PC) manifest impaired glucose homeostasis. While dietary therapies are sufficiently successful for patients to attain near normal growth and pubertal development, long-term complications, including hepatocellular adenoma (HCA) with malignant potential, persist in GSD-Ia patients. Using G6pc−/− (GSD-Ia) mice, we examined the efficacy of liver G6Pase-α delivery mediated by rAAV8-GPE, an recombinant AAV serotype 8 vector expressing human G6Pase-α directed by the human G6PC promoter/enhancer (GPE), and showed that rAAV8-GPE–mediated gene transfer completely normalizes hepatic G6Pase-α deficiency for at least 24 weeks. A remaining concern with the therapy is the risk of HCA. A recent study showed that HCA develops in 100% of liver-specific G6pc-null mice 78 weeks after gene deletion. Therefore, we examined the disease risk for hepatic neoplasia in a long-term study using G6pc−/− mice. We showed that rAAV8-GPE–mediated gene therapy maintains efficacy for at least 70–90 weeks for mice expressing more than 3% of wild-type hepatic G6Pase-α activity. The treated mice displayed normal hepatic fat storage, normal blood metabolite and glucose tolerance profiles, and reduced fasting blood insulin levels and maintained normoglycemia over a 24-hour fast. Ultrasound and histological examinations demonstrated neither hepatic steatosis nor detectable HCA or hepatocellular carcinoma in the liver of any of these mice. After a 24-hour fast, hepatic G6PT mRNA levels in rAAV8-GPE–treated G6pc−/− mice were markedly elevated. The fact that G6PT transport is the rate-limiting step in microsomal G6P metabolism may explain why the treated G6pc−/− mice could sustain prolonged fasts. The low fasting blood insulin levels and lack of hepatic steatosis may explain the absence of HCA. Our results suggest that G6Pase-α gene transfer may offer a therapeutic approach to the management of human GSD-Ia.
The upstream enhancer elements of the G6PC promoter are critical for optimal G6PC expression.
The most efficacious gene therapy vectors for GSD-Ia reported to date are rAAV8-GPE, a single-stranded (ss) vector containing a 2864-bp of the G6PC promoter/enhancer (GPE) and rAAV8-miGPE, a double-stranded (ds) vector containing a shorter 382-bp minimal GPE. Studies have shown that the ds rAAV vectors possess an increased transduction efficiency, which arises from bypassing the rate-limiting conversion of ss to ds vector genomes during transduction. However, the trade-off of the ds rAAV vector is a 50% lower packaging capacity than in the ss rAAV vector, which restricts the size of the promoter/enhancer it can carry, such as the rAAV8-miGPE vector. To determine the best vector to take forward into clinical trials, we initiated a direct comparison of the rAAV8-GPE and the rAAV8-miGPE vectors. We showed that the rAAV8-GPE vector directed significantly higher levels of hepatic G6Pase-α expression, achieved greater reduction in hepatic glycogen accumulation, and led to improved toleration of fasting in GSD-Ia mice than did the rAAV8-miGPE vector. Our results indicated that additional control elements in the ss rAAV8-GPE vector outweigh the gains from the ds rAAV8-miGPE transduction efficiency and that the rAAV8-GPE vector should be the current choice for clinical translation in human GSD-Ia.
SLC37A1 and SLC37A2 are phosphate-linked G6P antiporters.
G6PT belongs to the SLC37 family of membrane-bound transporters. Only G6PT, also known as SLC37A4, has been characterized, and it acts as a phosphate (Pi)-linked G6P antiporter. The other three SLC37 family members, predicted to be sugar-phosphate:Pi exchangers, have not been characterized functionally. We therefore investigated whether any of these proteins might also have a G6P transport activity. Using reconstituted proteoliposomes, we examined the antiporter activity of the other SLC37 members along with their ability to couple with G6Pase-α. We showed that SLC37A1 and SLC37A2 are ER–associated, Pi-linked antiporters that can transport G6P. The activity of SLC37A3 is unknown. Unlike G6PT, neither is sensitive to chlorogenic acid, a competitive inhibitor of physiological ER G6P transport, and neither couples to G6Pase-α. We conclude that three of the four SLC37 family members are functional sugar-phosphate antiporters. However, only G6PT/SLC37A4 has the characteristics of the physiological ER G6P transporter, suggesting that the other SLC37 proteins play roles that are independent of blood glucose homeostasis.
Molecular mechanisms of neutrophil dysfunction in GSD-Ib
The underlying cause of GSD-Ib neutropenia is enhanced neutrophil apoptosis, but patients also manifest neutrophil dysfunction of unknown etiology. Previously, we showed that G6PT interacts with the enzyme G6Pase-β to regulate the availability of G6P/glucose in neutrophils. Deficiency in G6Pase-β activity in neutrophils impairs both their energy homeostasis and functionality. Recently, we showed that G6PT–deficient neutrophils from GSD-Ib patients are similarly impaired. Their energy impairment is characterized by reductions in glucose uptake and in levels of intracellular G6P, lactate, ATP, and NADPH, while functional impairment is reflected in reduced neutrophil respiratory burst, chemotaxis, and calcium mobilization. Moreover, we show that the hypoxia-inducible factor-1α (HIF-1α)/peroxisome proliferators-activated receptor-γ (PPAR-γ) pathway is activated in G6PT–deficient neutrophils, leading to impairment in neutrophil respiratory burst, chemotaxis, and calcium mobilization activities. Taken together, the findings show that the G6PT–mediated G6P/glucose cycling is essential for neutrophil homeostasis and G6P metabolism and that a deficiency leads to impaired energy homeostasis. We further showed that the mechanism of neutrophil dysfunction in GSD-Ib arises from activation of the HIF-1α/PPAR-γ pathway.
Additional Funding
- The Childrens' Fund for Glycogen Storage Disease Research, 2012, ongoing
Publications
- Pan CJ, Chen SY, Jun HS, Lin SR, Mansfield BC, Chou JY. SLC37A1 and SLC37A2 are phosphate-linked, glucose-6-phosphate antiporters. PloS One 2011;6(9):e231.
- Jun HS, Cheung YY, Lee YM, Mansfield BC, Chou JY. Glucose-6-phosphatase-β, implicated in a congenital neutropenia syndrome, is essential for macrophage energy homeostasis and functionality. Blood 2012;119:4047-4055.
- Lee YM, Jun HS, Pan CJ, Lin SR, Wilson LH, Mansfield BC, Chou JY. Prevention of hepatocellular adenoma and correction of metabolic abnormalities in murine glycogen storage disease type Ia by gene therapy. Hepatology 2012;56:1719-1729.
- Chou JY, Jun HS, Mansfield BC. The SLC37 family of phosphate-linked sugar phosphate antiporters. Mol Aspects Med 2012;34:601-611.
- Lee YM, Pan CJ, Koeberl DD, Mansfield BC, Chou JY. The upstream enhancer elements of the G6PC promoter are critical for optimal G6PC expression in murine glycogen storage disease type Ia. Mol Genet Metab 2013;110:275-280.
Collaborators
- Barry J. Byrne, MD, University of Florida College of Medicine, Gainesville, FL
- Thomas Conlon, PhD, University of Florida College of Medicine, Gainesville, FL
- Alessandra Eva, PhD, Gaslini Institute, Genova, Italy
- Luigi Varesio, PhD, Gaslini Institute, Genova, Italy
- David A. Weinstein, MD, University of Florida College of Medicine, Gainesville, FL
Contact
For further information, contact chouja@mail.nih.gov.