You are here: Home > Section on Chromatin and Gene Expression
Chromatin Remodeling and Gene Activation
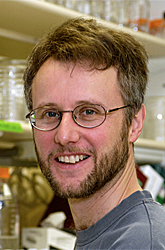
- David J. Clark, PhD, Head, Section on Chromatin and Gene Expression
- Hope A. Cole, PhD, Postdoctoral Fellow
- Peter Eriksson, PhD, Research Fellow
- Dwaipayan Ganguli, PhD, Visiting Fellow
- Nagarajavel Vivekananthan, PhD, Visiting Fellow
Gene activation involves the regulated recruitment of factors to a promoter in response to appropriate signals, ultimately resulting in the formation of an initiation complex with RNA polymerase II and then transcription. These events must occur in the presence of nucleosomes, which are compact structures capable of blocking transcription at every step. To circumvent the chromatin block, eukaryotic cells possess chromatin-remodeling and nucleosome-modifying complexes. The former (e.g., SWI/SNF complexes) use ATP to drive conformational changes in nucleosomes and to slide nucleosomes along DNA. The latter contain enzymatic activities (e.g., histone acetylases) that modify the histones post-translationally to mark them for recognition by other complexes. Geneticists have described many interesting connections between chromatin components and transcription, but they have lacked a system to investigate the structural basis of the connections. We have developed such a model system, involving native plasmid chromatin purified from the yeast cerevisiae, to perform high-resolution studies of the chromatin structures of active and inactive genes. Remarkably, our work reveals that activation correlates with large-scale movements of nucleosomes and conformational changes within nucleosomes over entire genes. Our current work involves two major projects: in the first, we are taking advantage of the recent technological breakthrough in DNA sequencing (high-throughput sequencing) to determine whether our observations concerning gene activation in chromatin can be extrapolated to the entire yeast genome; in the second, we are addressing the role of a sequence-specific DNA-binding histone acetylase, Spt10p, in the cell cycle–dependent regulation of the histone genes.
Transcriptional activation and SWI-/SNF-dependent nucleosome mobilization
We chose budding yeast as a model organism because biochemical studies of chromatin structure could be combined with molecular genetics. Current models for the role of the SWI/SNF ATP-dependent chromatin remodeling complex in gene regulation focus on promoters, where the most obvious changes in chromatin structure occur. However, using our plasmid model system with HIS3, a SWI/SNF–regulated gene, we discovered that transcriptional activation creates a domain of remodeled chromatin structure that extends far beyond the promoter, to include the entire gene. We addressed the effects of transcriptional activation on the chromatin structure of HIS3 by mapping the precise positions of nucleosomes in basal expressing and transcriptionally activated chromatin. In the absence of the Gcn4p activator, the HIS3 gene is organized into a dominant nucleosomal array. In wild-type chromatin, this array is disrupted, and several alternative, overlapping, nucleosomal arrays are formed. Disruption of the dominant array also requires the SWI/SNF remodeling machine, indicating that the SWI/SNF complex plays an important role in nucleosome mobilization. We propose that Gcn4p stimulates nucleosome mobilization over the entire HIS3 gene by the SWI/SNF complex. We suggest that the net effect of interplay among remodeling machines at HIS3 is to create a highly dynamic chromatin structure (Figure 1). Our work on HIS3 and our earlier work on CUP1 indicate that, at least for these two genes, the target of remodeling complexes is a domain rather than just the promoter. This is an important finding, because it suggests that remodeling complexes act on chromatin domains. As to the function of domain remodeling, we speculate that remodeling entire genes might facilitate elongation through nucleosomes by RNA polymerase II.
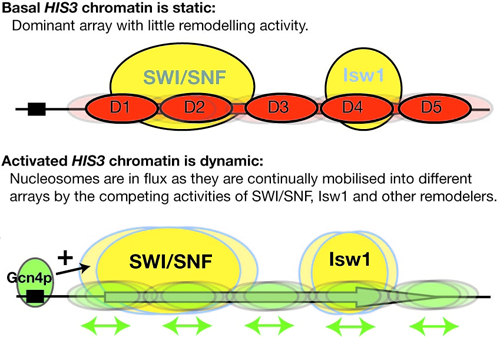
Figure 1. Working model for activation of the HIS3 gene
Basally expressed HIS3 exists in a relatively static chromatin structure approximated by a single ordered nucleosomal array (D1-D5). Activation by Gcn4p initiates nucleosome mobilization by the SWI/SNF and Isw1 complexes. It is suggested that this creates a dynamic chromatin structure in which nucleosomes are shunted back and forth, from one array to another. This would render the DNA transparent to transcription initiation and elongation factors. Not shown: In activated chromatin, nucleosome structure is also remodeled in a SWI/SNF– and Isw1–dependent process.
The questions that form the basis of our current work concern: (1) the structure of the remodeled nucleosome; there are at least two possibilities: unstable nucleosomes (remodeled such that they fall apart easily) and nucleosomes with a dramatically altered conformation; and (2) how general chromatin domains are remodeling in yeast. We are mapping nucleosomes on a genome-wide scale using high-throughput sequencing.
A nucleosomal barrier to transcription by RNA polymerase II in vitro and in vivo
Synthetic nucleosome positioning sequences such as 601 and 603 have been invaluable for experiments in vitro in which a uniquely positioned nucleosome is required. Previously, the Studitsky Lab showed that the 603-nucleosome acts as a polar barrier to transcription by RNA polymerase II in vitro. To test whether the 603-sequence is also a polar block to transcription in vivo, we inserted the 603-sequence between the transcription and translation start sites of the copper-inducible yeast CUP1 gene. We are determining the effects of the 603-sequence on CUP1 transcription and whether the 603-nucleosome is formed as expected in vivo. We expect to gain insight into the role of nucleosomes in the control of transcript elongation by RNA polymerase II.
The yeast Spt10 protein contains a DNA-binding domain fused to a putative histone acetylase domain
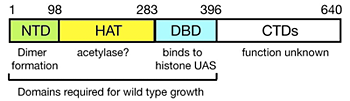
Figure 2. Domain structure of Spt10p
NTD = N-terminal domain; HAT = histone acetylase; DBD = DNA-binding domain; CTDs = C-terminal domains; UAS = upstream activating sequences.
We have shown previously that induction of CUP1 by copper results in targeted acetylation of nucleosomes at the CUP1 promoter. This acetylation is dependent on SPT10, which encodes a putative histone acetylase (HAT). SPT10 has been implicated as a global regulator of core promoter activity. Using expression microarray analysis, we confirmed that Spt10p has global effects on transcription. We addressed the mechanism of global regulation. Surprisingly, we were unable to detect Spt10p at any of the most strongly affected genes in vivo using the chromatin immunoprecipitation (ChIP) assay. However, we confirmed that Spt10p is present at the core histone gene promoters, which it activates. We found evidence that a defective chromatin structure is formed in the absence of Spt10p, with consequent activation of basal promoters. Furthermore, we found that Spt10p binds specifically and highly cooperatively to pairs of upstream activating sequences (UAS elements) in the core histone promoters (consensus: (G/A)TTCCN6TTCNC), consistent with a direct role in histone gene regulation. No other high-affinity sites are predicted in the yeast genome. Our observations are consistent with the idea that the global changes in gene expression observed in spt10-null cells are actually the indirect effects of defective regulation of the core histone genes. Thus, Spt10p is a sequence-specific activator of the histone genes, possessing a DNA-binding domain (DBD) fused to a likely HAT domain, rather than to a classical activation domain. Spt10p is therefore a very unusual trans-activator, in which the HAT domain, normally recruited as a co-activator to promoters through an activation domain, is attached directly to a sequence-specific DNA-binding domain (Figure 2).
We identified the DNA binding domain (DBD) of Spt10p: it contains an unusual zinc finger (His2-Cys2), which has homology to the DNA integrase of foamy retroviruses. We proposed that this integrase might also be a sequence-specific DNA-binding protein. To test this hypothesis, we initiated a new project to determine whether human foamy virus (HFV) integrase is indeed a sequence-specific DNA-binding protein, using a SELEX approach.
We have addressed the mechanism through which Spt10p recognizes two UAS elements simultaneously. We demonstrated that Spt10p is an elongated dimer and that its N-terminal domain is necessary for dimer formation. The isolated DBD is a monomer and binds tightly to a single UAS element, unlike the full-length protein dimer, which requires two UAS elements. We propose that the Spt10p dimer undergoes a major conformational change in order to recognize two UAS elements at the same time.
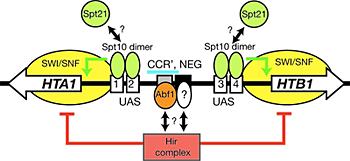
Figure 3. Regulation of the HTA1-HTB1 locus
A Spt10p dimer binds at each pair of UAS elements and activates transcription when a cell cycle–regulated signal is received (which could be Spt21p, or phosphorylation by a cell cycle–dependent kinase). Activation requires SWI/SNF and is repressed by the Hir complex; both effects require the NEG region (blue bar), which contains CCR′ (an Abf1p site) and part of the NEG element (black box), which might bind to another protein.
Our current work has the following aims:
- Demonstrate the putative histone/protein acetylase activity of Spt10p; we are using a proteomics approach.
- Understand the role of Spt10p in the cell cycle–dependent regulation of the core histone genes (Figure 3); the cell-cycle transcription factor SBF is a heterodimer of Swi4p and Swi6p, Swi4p contains the sequence-specific DBD, and Swi4-binding sites are predicted in the HTA1-HTB1 promoter; these overlap three of the four UAS elements; we are exploring the possibility that SBF and Spt10p compete for binding to the UAS elements.
- Identify negative regulatory proteins at the histone promoters, which might counteract activation by Spt10p; we have identified candidates and are currently validating them.
Publications
- Clark DJ, Leblanc B. Analysis of DNA supercoiling induced by DNA-protein interactions. Meth Mol Biol 2009 543:523-535.
Collaborators
- Vasily M. Studitsky, PhD, University of Medicine and Dentistry of New Jersey, Piscataway, NJ
Contact
For more information, email clarkda@mail.nih.gov or visit ucge.nichd.nih.gov.