You are here: Home > Section on Intracellular Protein Trafficking
Protein Sorting in the Endosomal-Lysosomal System
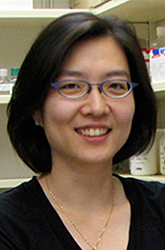
- Sohyun Ahn, PhD, Head, Unit on Developmental Neurogenetics
- Andrea Barrett, PhD, Postdoctoral Fellow
- Guannan Ge, BS, Postbaccalaureate Fellow
- Lindsay Hayes, BS, Predoctoral Fellow: Brown-NIH Graduate Partnerships Program
- Sherry Ralls, BA, Technician-Biologist
- Hui Wang, PhD, Visiting Fellow
The laboratory continues to investigate the role of Sonic hedgehog (Shh) and its downstream signaling components in the developing and mature nervous system. We have demonstrated a requirement for Gli3, a major negative regulator of the Shh pathway, in intermediate progenitors during embryonic cortical development. We are currently investigating the role of Shh downstream effectors in adult neurogenesis using conditional gene ablation approaches in mouse. In addition, we have identified several genes that are specifically expressed in adult neural stem cells, and we are in the process of validating their expression and function. We are also investigating the distinct spatial and temporal contribution of Shh and Gli1 lineage cells in dopaminergic neuron development in the ventral midbrain to better understand the origin of diversity among dopamine neurons and their circuit formation. Our studies will provide the foundation needed for stem cell biology to develop therapeutic methods for treating various neurodegenerative diseases.
The role of Gli3 in embryonic neurogenesis
The Shh signaling pathway is transduced via two major effectors: Gli2 as an activator, and Gli3 as a repressor following a post-translational modification. Given that Gli3 null mice have severe neural patterning defects and die at birth, we used a conditional gene ablation approach with the pan-neuronal deleter mouse Nestin-Cre to address the role of Gli3 in later neural developmental processes such as corticogenesis and differentiation. First, we found that the generation of intermediate progenitors during cortical development is greatly disrupted in the absence of Gli3, resulting in a thinner neocortex with the more pronounced defects occurring in the superficial cortical layers II/III. As a consequence, the corpus callosum, which consists mainly of axonal projections emanating from the layers II/III neurons, is greatly reduced in the postnatal forebrain of the Gli3 conditional mutants. Furthermore, using electroporation, we confirmed the cell-autonomous requirement for Gli3 in specification and terminal differentiation of callosal projecting neurons. Specifically, Gli3 mutant cells in the wild-type environment were generated, but failed to migrate normally to the most superficial layer of the neocortex and to express the appropriate molecular markers of terminally differentiated cortical neurons of layers II/III. Taken together, our results demonstrate the importance of Gli3, the negative regulator of Shh signaling, for the generation of intermediate progenitors during embryonic neurogenesis and, ultimately, for proper cortical neuron specification.
The role of Gli2 or Gli3 in the establishment and maintenance of adult NSCs and their niche
The adult forebrain continuously generates new neurons in two discrete regions, the subventricular zone (SVZ) of the lateral ventricle and the subgranular zone of the dentate gyrus in the hippocampus. By the end of embryonic neurogenesis in rodents, Radial Glial Cells (RGCs) become postnatal neural stem cells (NSCs) and ependymal cells. Interestingly, these RGC-like cells, which express NSC markers, persist in postnatal forebrains of Gli3 conditional mutants while the ependymal cells lining the wall of the lateral ventricle are greatly misshapen and misplaced. Further molecular marker analysis reveals that the cytoarchitecture of the SVZ is severely disrupted: NSCs and neuroblasts appear in clusters and protrude directly to the lumen of the lateral ventricle. As a consequence of defects in proper cell-type transition and migration, the olfactory bulb is markedly reduced in size. We are currently investigating the molecular mechanism by which Gli3 affects the establishment of NSCs. In addition, we plan to dissect the role of Gli3 specifically in the ependymal cells compared with NSCs in the SVZ by conditionally ablating Gli3 with FoxJ1-Cre (ependymal-specific deletion) or GFAP-Cre mice (NSCs and astrocytes). To investigate the role of Gli3 or Gli2 in the maintenance of NSCs in postnatal brains, we have begun to analyze conditional mutants with a specific ablation of Gli2 or Gli3 in Shh-responding NSCs using Gli1-CreER mice.
Gene expression profiling of adult neural stem cells and their niche
We have isolated Shh-responding NSCs based on their expression of Gli1 and GFAP, using fluorescence-activate cell sorting (FACS) from two neurogenic regions of the forebrain: the SVZ of the lateral ventricle and the dentate gyrus (DG) of the hippocampus. Affymetrix microarray experiments enabled us to compare gene expression profiles of the SVZ and hippocampus with the cerebellum, a non-neurogenic region where the Gli1- and GFAP-expressing cells are Bergman Glia cells. Our initial analysis indicates that the overall profiles of the neurogenic SVZ and hippocampus are significantly different from that of the non-neurogenic cerebellum. In brief, we found 66 genes and 125 genes to be differentially expressed between hippocampus and SVZ, respectively, when compared with the cerebellum. Among these distinct genes, 16 genes are expressed in both the SVZ and hippocampus. We are currently validating the gene expression profiles using RT-PCR and RNA in situ hybridization. We will investigate the functional significance of the validated genes in NSC biology in vitro and in vivo.
In addition, we are comparing the gene expression profiles of NSCs with those of the putative neurogenic niche cells, including endothelial cells in the SVZ, astrocytes, and ependymal cells. Given that the behavior of NSCs is dictated by extrinsic cues provided in their niche, we are specifically interested in signaling molecules such as secreted ligands and their receptors and extracellular cell-cell contact molecules. Using various genetically modified mouse lines and fluorescent reporter lines, we plan to isolate each cell population using FACS and perform gene expression profiling using the Signal Sequence Trapping (SST) method. We have validated the specificity of the cell types labeled in these various mouse lines using immunohistochemistry and are currently isolating specific cell populations using FACS. Compared with microarray experiments designed to identify specific genes downstream of Shh signaling, this alternative SST method demonstrates possible interacting components (either through secretion or cell-cell contact) between NSCs and their putative niches in an unbiased manner.
Hippocampal neurogenesis
Another neurogenic region in the adult forebrain is the subgranular zone (SGZ) of the DG in the hippocampus. New granule neurons are continuously generated and integrated into the existing neural circuits. As in the SVZ of the lateral ventricle, NSCs in the SGZ of the DG also respond to Shh signaling. We have investigated when Shh-responding NSCs are established in the hippocampus by profiling the expression patterns of Shh and its response gene, Gli1, starting at E18.5 to neonatal stages, using Shh-Cre:GFP and Gli1-nLacZ mice. We found that the Shh-responding (Gli1-expressing) cells start to appear in the DG at the late gestational stage and increase in number in the neonatal stages. However, the location of Shh-responding cells is restricted to the SGZ, and they are not found in other hippocampal structures. In contrast, Shh-expressing cells first appear in the hilus region of the dentate gyrus and CA3 region of the hippocampus at E18.5 and persist in postnatal stages. Interestingly, cumulative fate mapping of the Shh-lineage (Shh-Cre; TaumGFP) reveals that cells expressing Shh do not change in number or send projections that innervate the molecular layer of the DG. In contrast, Shh-responding cells increase in number as the NSCs proliferate and generate more granule neurons, which mature over time to innervate CA3 pyramidal neurons. We are in the process of characterizing the molecular nature of the Shh- and Gli1-lineage cells using immunohistochemistry.
The genetic lineage of midbrain dopaminergic neurons
Dopaminergic (DA) neurons in the ventral midbrain are diverse in their spatial distribution and neural circuits, ultimately resulting in various physiological functions. We are currently investigating the origin of diversity among midbrain DA neurons by analyzing the contribution of two genetic lineages: Shh and Gli1. Temporal fate mapping using Shh-CreER and Gli1-CreER mice show that the Shh- and Gli1-lineage cells contribute to somewhat distinct populations of DA neurons along the medial/lateral and anterior/posterior axes. This study will be extended to the postnatal mature brain to investigate the neural circuitry established by these two genetic lineages that derive from distinct developmental periods.
In addition, we have begun gain-of-function and loss-of-function experiments using ROSA26loxP-STOP-loxP-SmoM2 and SmoFlox mice, respectively, to perturb Shh signaling in distinct spatial and temporal domains to address the requirement of Shh signaling in DA neuron diversity.
Collaborators
- Mark Zervas, PhD, Brown University, Providence, RI
- Yosuke Mukoyama, PhD, Laboratory of Developmental Biology, NHLBI, Bethesda, MD
Contact
For more information, email ahnsohyun@mail.nih.gov or visit http://neuroscience.nih.gov/Lab.asp?Org_ID=542.