You are here: Home > Section on Formation of RNA
Physiological, Biochemical, and Molecular Genetic Events of Recognition and Resolution of RNA/DNA Hybrids
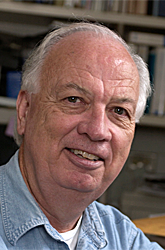
- Robert J. Crouch, PhD, Head, Section on Formation of RNA
- Susana M. Cerritelli, PhD, Staff Scientist
- Hyongi Chon, PhD, Postdoctoral Fellow
- Lina Gugliotti, PhD, Postdoctoral Fellow
- Mitsuru Haruki, PhD, Guest Researcher
- John B. Holmes, BS, Predoctoral Fellow, NIH/Oxford/Cambridge Graduate Partnerships Program
- Kiran Sakhuja, MS, MSc, Research Assistant
- Yutaka Suzuki, PhD, Postdoctoral Fellow
Damaged DNA is one of the leading causes of many human diseases and disorders. Our studies are directed toward the formation and resolution of RNA/DNA hybrids, which occur during DNA and RNA synthesis. Such hybrid molecules may lead to increased DNA damage but may also play critical roles in normal cellular processes. We are interested in understanding how RNA/DNA hybrids are resolved and what role ribonucleases H (RNases H) play in their elimination. Two classes of RNases H are present in most organisms. Our studies have shown that mice deleted for the Rnaseh1 gene arrest embryonic development at day 10 due to a failure to amplify mitochondrial DNA. Others have found that Aicardi-Goutières Syndrome (AGS), a severe neurological disorder with symptoms appearing at or soon after birth, can be caused by defective human RNase H2. We employ molecular-genetic and biochemical tools and yeast and mouse models in our research.
RNA/DNA hybrids can be composed of one strand RNA and a second strand DNA or can be in the form of an R-loop in which the two DNA strands are separated, with only one hybridized to RNA while the other is single-stranded DNA. Both types of hybrid are substrates for the two classes of RNases H. Simple (non-R-loop) RNA/DNA hybrids are formed when the HIV-AIDS virus copies its genomic RNA into DNA using a reverse transcriptase (RT). The RT also has an RNase H domain that is structurally and functionally similar to the class I cellular RNase H and is necessary in several steps of viral DNA synthesis. It is known that during RNA synthesis R-loops can form and that aberrant R-loop formation can result in chromosome breakage. However, R-loop formation has been observed in the normal recombination process of switching (recombination) from one form of immunoglobulin to another resulting in different isoforms of antibodies.
Structure-function of ribonucleases H: RNase H1
RNA/DNA hybrids are essential intermediates in the replication of HIV’s RNA genome. In addition, the hybrids are believed to be necessary for mitochondrial DNA replication and important for switching immunoglobulin isotypes (e.g., from IgM to IgA). How these enzymes recognize and cleave the RNA is important for our understanding of the biology of these diverse events and for possible regulation of RNase H activity. Bacterial RNases HI are generally small proteins (150 amino acids) that share significant similarity with their eukaryotic counterparts in structure, interaction with their substrates, and the mechanism of cleavage. The protein structure of E. coli RNase HI unbound to a substrate is similar to that of the structure of human RNase H1 in complex with an RNA/DNA hybrid. The enzyme recognizes at least four hydroxyl groups of the ribose, with the DNA significantly distorted so that one phosphate is able to bind in a pocket on the enzyme (Nowotny et al., Cell 2005;121:1005). Both properties contribute to the specificities of the enzyme. The “basic protrusion” of the enzyme has extensive interactions with the hybrid, adding to the stability of the complex. Eukaryotic RNases H have an N-terminal domain (absent from the bacterial enzyme) that binds to RNA/DNA, conferring processivity to the enzyme (Gaidamakov et al., Nucleic Acids Res 2005;33:2166). This N-terminal domain (HBD) interacts with RNA/DNA through contacts with both the DNA and RNA strands and may provide the initial contact between the enzyme and hybrid.
The N- and C-terminal domains are connected by 65 amino acids in the human enzyme and by 64 in the mouse enzyme. The length and sequence of the connection domain are extremely variable in other species, suggesting that RNase H activity may not depend on any particular amino acid number or sequence. Our studies indicate that the connection domain is important for flexibility, allowing the protein to bind and cleave more effectively. Our current model of RNase H1 action posits that binding of the enzyme occurs mainly via the HBD and that the RNase H domain searches for an appropriate cleavage site and cleaves; following release of the RNase H domain from the hydrolyzed substrate, the HBD anchors the protein while the RNase H domain recognizes another site and cleaves; the process continues until either the HBD releases from the hybrid or no more RNase H cleavage sites are available to be attacked.
Functions of RNase H1 in mitochondria and nuclei
One of the major challenges we face in understanding the roles of RNase H1 in cells is to determine how the translation of a single mRNA can produce both nuclear and mitochondrial forms of the enzyme. Knocking out the Rnaseh1 gene in mouse results in embryonic lethality at embryonic day 8.5 due to a failure to replicate mitochondrial DNA. The outcome indicates both that the enzyme is essential for the maintenance of mtDNA and that there is no need for newly synthesized RNase H1 for replication/repair of nuclear DNA.
Translation of the single Rnaseh1 mRNA initiates at two distinct start codons, with the resulting proteins being targeted to either the mitochondria or the nucleus. Our current studies suggest that the level of each protein is affected by a short upstream open reading frame. Thus, the amount of protein(s) is (are) kept at low levels by differential translation. This finding suggests that the very low amount of Rnaseh1 mRNA observed in most cells is more than sufficient to generate the quantity of RNase H1 that is optimal and that relatively poor translation is used to limit the amount of enzyme produced. We have generated transgenic mice that express elevated levels of RNase H1 in B-cells; the increase results in no obvious change in mitochondria. We are examining effects of even higher levels of the nuclear form of RNase H1 on B cells, where recombination of the immunoglobulin locus results first in joining of the VDJ regions and then later in B-cell development when isotype switching occurs. These events involve numerous proteins used during repair of damaged DNA.
We have generated a conditional knockout that permits us to determine whether RNase H1 is necessary in adult animals either for mtDNA or nuclear DNA replication and repair. It may be that RNase H1 is important for mtDNA during embryogenesis only when, after implantation, rapid mtDNA synthesis occurs, at which time synthesis is suddenly activated. In addition, we will be able to generate organ-/tissue-specific knockouts of the Rnaseh1 gene by using the Cre-lox system, including Tamoxifen®-inducible Cres for general ablation and others (e.g., heart-specific Cre expressers). However, the problem of inactivating the synthesis of both forms remains unresolved. Accordingly, we have generated transgenic mice that produce either both isoforms of RNase H1 or only the nuclear form in T and B cells.
In collaboration with Ian Holt, we are searching for possible roles of RNase H1 in mitochondrial DNA replication by using, among other techniques, analysis of intermediates on two-dimensional gels. Our findings thus far indicate that elevated expression of RNase H1 in mitochondria alters mtDNA replication. In addition, data obtained in Holt’s laboratory by John Holmes supporting the existence of RNA/DNA hybrids as replication intermediates indicate a bi-directional mode of DNA replication for this organelle.
Structure-function of ribonucleases: RNase H2
We previously found that, in Saccharomyces cerevisiae, RNase H2, the second type of RNase H, is composed of three subunits (Jeong et al., Nucleic Acids Res 2004;32:407), two of which we did not find in higher eukaryotes when using a BLAST search. We determined that there are three subunits of RNase H1 in human cells and were able to find similar RNases H2 in other mammals, in particular the mouse. A recent paper in Nature Genetics reported that mutations in any one of these proteins leads to the rare Aicardi-Goutières syndrome (AGS). Our studies on the human and yeast enzymes indicate a connection between RNase H2 and PCNA (proliferating cell nuclear antigen)—a clamp-loader protein involved in recruiting proteins to DNA for repair and replication. A PCNA-Interacting-Peptide (PIP) is present in the RNase H2B subunit of yeast, mouse, and human RNases H2. Using several types of analyses, we demonstrated that the PIP of the RNase H2B is indeed able to interact with PCNA, a finding whose biological significance we are continuing to explore. We also examined the effects of several of the AGS-causing mutations on recombinant RNase H2 activity. Only two of the mutant enzymes have shown decreased activity. The other altered proteins are most likely defective in vivo for complex formation and/or stability.
Publications
- Nowotny M, Cerritelli SM, Ghirlando R, Gaidamakov SA, Crouch RJ, Yang W. Specific recognition of RNA/DNA hybrid and enhancement of human RNase H1 activity by HBD. EMBO J 2008 27:1172-1181.
- Rohman MS, Koga Y, Takano K, Chon H, Crouch RJ, Kanaya S. Effect of the disease-causing mutations identified in human RNase H2 on the activities and stabilities of yeast RNase H2 and archaeal RNase HII. FEBS J 2008 275:4836-4849.
- Chon H, Vassilev A, DePamphilis ML, Zhao Y, Zhang J, Burgers PM, Crouch RJ, Cerritelli SM. Contributions of the two accesory subunits, RNASEH2B and RNASEH2C, to the activity and properties of the human RNase H2 complex. Nucleic Acids Res 2009 37:96-110.
- Cerritelli SM, Crouch RJ. Ribonuclease H: the enzymes from eukaryotes. FEBS J 2009 276:1494-1505.
Collaborators
- Peter Burgers, PhD, Washington University, St. Louis, MO
- Ian Holt, PhD, MRC-Dunn Nutrition Unit, Cambridge, UK
- Shigenori Kanaya, PhD, Osaka University, Osaka, Japan
- Paul E. Love, MD, PhD, Program in Genomics of Differentiation, NICHD, Bethesda, MD
- Marcin Nowotny, PhD, International Institute of Molecular and Cell Biology, Warsaw, Poland
- Herbert C. Morse, MD, Laboratory of Immunopathology, NIAID, NIH, Bethesda,MD
- Wei Yang, PhD, Laboratory of Molecular Biology, NIDDK, Bethesda, MD
Contact
For more information, email crouch@helix.nih.gov or visit sfr.nichd.nih.gov.