You are here: Home > Section on Molecular Regulation
Global Regulation of Bacterial Gene Expression by ppGpp
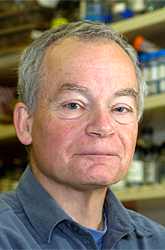
- Michael Cashel, MD, PhD, Head, Section on Molecular Regulation
- Christophe Penno, PhD, Visiting Fellow
- Katarzyna Potrykus, PhD, Research Fellow
- Daniel Vinella, PhD, Research Scholar
In apparent defiance of the usual rules of regulatory specificity, the bacterial global regulation signal ppGpp can sense limitation for almost any nutrient (nitrogen, carbon, amino acids, some vitamins, phosphate, iron, or oxygen) as well some sources of stress (membrane, pH, osmotic, or heat). The guanine nucleotide analog ppGpp is found in almost all bacteria and plants. The response to the ppGpp signal has been most extensively studied in E. coli, where it up- or down-regulates the transcription of more than 1,300 genes, one third of the genome. Regulation occurs by direct ppGpp effects on RNA polymerase, by integrating ppGpp regulatory effects with effects of other regulators, and through effects on alternative sigma factors. Regulatory effects of ppGpp in Gram-positive bacteria are less well studied but the available evidence already shows similarities (inhibition of rRNA synthesis) as well as differences (inhibition of DNA primase). In all bacteria, the molecular details of how ppGpp works are still uncertain. Both the breadth of conditions that provoke ppGpp and its mechanism of action contrasts with those of cAMP, another modified purine nucleotide. The cAMP second messenger senses only glucose availability and responds with more limited regulatory effects.
Most is known about the inhibitory effects of ppGpp that occur in E. coli by direct inhibition of transcription by RNA polymerase; the mechanism is atypical for bacterial transcription regulation because ppGpp regulation does not involve recruitment of RNA polymerase by a DNA sequence–recognition protein, as is the case for cAMP with cAMP receptor protein. Instead, ppGpp directly interacts with RNA polymerase, sometimes requiring additional transcription factors. The expertise of our laboratory centers around ppGpp and its effects on transcription. We focus on clarifying fundamental roles of ppGpp activating transcription in microbial physiology because many reports in the literature in the past few years indicated that ppGpp is required for pathogenicity and/or the transcription of virulence genes on pathogenicity islands. The list of pathogens with these features is broad and includes: Bacillus anthracis (anthrax); Brucella melitensis (brucellosis); Borelia burgdorferi (Lyme disease); Camplobacter jejuni (toxic diarrhea); Enterobacter jejuni (diarrhea); E. coli, (meningitis, hemorrhagic toxicity); Helicobacter pylori (peptic ulcer); Legionella pneumophilia (Legionaires disease); Mycobacteria tuberculosis (tuberculosis); Pseudomonas aeruginosa (pneumonia); Streptococcus mutans (caries); Streptococcus pneumonia (pneumonia); Salmonella typhimurium (typhoid); Vibrio cholerae (cholera); and Yersinia pestis (plague). The ppGpp requirement for bacterial virulence is so strong that, in two instances with mouse models, pathogenicity was found attenuated by the absence of ppGpp to the extent of raising LD50 titers 10,000- to 100,000-fold (Y. pestis and S. typhimurium). These ppGpp-deficient strains were converted from lethal pathogens into effective live vaccines. During the past year, our transcription studies concentrated on positive regulation by ppGpp in E. coli, with a short-term goal to focus on mechanisms accounting for specific amino acid requirements (his, ile, leu, phe, ser, thr, val,) that appear when ppGpp is absent from E. coli. Our long-term goal is to understand the molecular basis of the ppGpp requirements for expression of virulence genes in bacterial pathogens in the hope of discovering applications that could lead to the development of new antibiotics or vaccines.
DksA, GreA, GreB, and TraR proteins unexpectedly affect positive regulation by ppGpp
Regulation of transcription by ppGpp at the molecular level is unusual (for prokaryotes) because it does not involve the typical interplay of sequence-specific DNA binding repressor or inducer proteins. Instead, ppGpp is thought to modify RNA polymerase initiation kinetics in ways that inhibit or activate specific classes of promoters, targeting core sequences. Regulation occurs by a synergistic interaction between ppGpp and DksA, a member of a group of structurally similar proteins called RNA polymerase secondary channel binding proteins. Other members of this group (GreA and GreB) can bind to the secondary channel in the absence of ppGpp and can alter phenotypes affected by DksA. These proteins can also reverse some of the phenotypes produced by the absence of both DksA and ppGpp. Examples include requirements for specific amino acids that arise in the absence of ppGpp and DksA. Evidently, under some experimental conditions, even in the absence of ppGpp, GreA/B can substitute for some regulatory functions of ppGpp on RNA polymerase. How might this work? Both GreA/B are well known to reverse arrest of transcription elongation that occurs when the 3’OH end of the nascent RNA is backtracked to distance itself from the catalytic center of RNA polymerase. However, DksA does not reverse arrest of transcription elongation. Our working hypothesis is that each of the secondary channel binding proteins can induce a conformational change in RNA polymerase without ppGpp, similar to the change induced by DksA and ppGpp. This conformational change leads to activation of the same spectrum of promoters that are activated by ppGpp and DksA.
It has been discovered that TraR should be added to the list of DksA-, GreA- and GreB-like proteins because of effects on phenotypes generated by a ppGpp deficiency. The traR gene is present on an F’ episomal element that mediates conjugation between male and female bacteria. The function of TraR does not involve conjugal transfer; TraR was so named because it is positioned between traQ and traS genes. Small amounts of TraR complement the amino acid requirements for growth in the absence of ppGpp. Under some conditions, GreA and GreB can also give similar growth phenotypes in the absence of ppGpp. TraR is also an inhibitor of ribosomal RNA transcription, like ppGpp. We demonstrated that TraR inhibition of rRNA promoters occurs in vitro with a pure transcription system in the absence of ppGpp, indicating a direct effect of TraR on transcription. The TraR sequence predicts that it might share with DksA, GreA, and GreB a structural feature—a finger postulated to be necessary for secondary channel penetration. However, the structural predictions for TraR are different from these three secondary channel binding proteins; TraR has only a single helical coil rather than a coiled-coil. Although it is plausible that this single-coil structure might work for secondary channel binding, secondary channel binding by TraR has not yet been demonstrated; the TraR structure only been predicted—not solved.
The discovery of GraL small RNA in the greA leader transcript arising from dual promoters
Our studies surveying regulation of greA expression have led to the finding that an apparently fixed portion of the greA leader transcripts are terminated before reaching the greA structural gene, so that the GreA protein is translated from transcripts that are not terminated. The leader termination event gives rise to a population of small RNA chains that we collectively call GraL. The GraL cluster contains terminated transcripts that end over a range of 12 nt. This extent of imprecise termination is very unusual for termination of both prokaryotic and eukaryotic transcripts, which usually differ over a range of only a few nt. Because of this novelty for GraL, we made a major effort to characterize the termination phenomenon. We documented that the GraL transcript chain lengths are variable because they actually have different 3′ hydroxyl ends. We showed that the GraL RNA chain clusters are formed in vitro with two different promoters (Sigma 70- and SigmaE-dependent). This indicates that the frayed ends are independent of the 5′ RNA chain start sites and not influenced by a specific sigma factor. Using immobilized transcription complexes, we proved that the GraL RNA chains are physically released from their immobilized state in elongation complexes. This proves that termination occurs with release and excludes the possibility that the GraL size distribution is due to transcription arrest. Genetic evidence with RNase gene deletions indicates that the frayed ends are not due to ribonuclease cleavage. The frayed ends reflect actual termination sites; this can be demonstrated in vitro with an array of antisense oligonucleotides to various portions of the abnormally long terminator stem-loop. These studies also define the lower portions of the stem loop that are determinants of incremental termination. GraL is not an in vitro artifact since the frayed ends are visualized by Northern blots with RNA preparations isolated from growing cells.
Surprisingly, the abundance of GraL itself does not seem itself to be regulated; if it were, an increase in GraL abundance would be associated with diminished GreA expression. Therefore the existence of GraL does not change cellular greA expression, raising the question of why GraL is present. Sequence comparisons among closely related bacteria reveal sequence divergence in the GraL region, yet RNA folding reveals that the terminator structures are conserved, evidence that favors evolutionarily conservation of the GraL termination function. We next investigated the biological importance of GraL by measuring a difference in fitness or selective advantage of GraL-overexpressing cells vs. cells not overexpressing GraL during repeated cycles of overnight growth in LB media. This was done by inoculating cultures with equal admixtures of cells containing either a GraL-overproducing plasmid (or vector) with one or the other marked with a malT mutant. The proportion of maltose-fermenting cells at any time of culture could be scored as the ratio of red and white colonies on maltose indicator plates. Cells overexpressing GraL were found to reproducibly and systematically out-compete wild-type cells whether or not the malT marker mutation was present together with the GraL plasmid. A scrambled GraL sequence control did not similarly affect fitness.
We also performed microarray analyses to search for target genes whose transcription is influenced by GraL. When GraL was overproduced for 15 minutes, no effect was found in wild-type strains. However, in the absence of ppGpp, over 100 genes were affected. Specifically, many genes affected by GraL are known to be repressed by Fur, a major regulator of iron metabolism. This is to be expected, given that many years ago we found that ppGpp responds to iron starvation. Flagellar genes also constitute a general class that is inhibited by GraL. Again, studies in our laboratory and those of others have implicated ppGpp and DksA in the regulation of flagellar genes. Computer searches that look for RNA-RNA hybrids as mediators of small RNA regulation did not identify mRNA targets that could be verified in the microarray results or by direct testing. Genetic evidence also excluded RNaseIII and Hfq as involved in regulation exerted by this small RNA. The results of this work indicate that GraL arises from a highly unusual series of termination events and that GraL has a biologically significant function. However, the specific targets and mechanisms of action for these targets have not yet been identified.
Publications
- Harinarayanan R, Murphy H, Cashel M. Synthetic growth phenotypes of E. coli lacking ppGpp and transketolase A (tktA) are due to ppGpp-mediated transcriptional regulation of tktB. Mol Microbiol 2008 69:882-894.
- Rhee H-W, Lee C-R, Cho S-H, Song M-R, Cashel M, Choy HE, Seok Y-J, Hong J-L. Selective fluorescent chemosensor for the bacterial alarmone (p)ppGpp. J Am Chem Soc 2008 130:784-785.
- Potrykus K, Cashel M. (p)ppGpp: still magical? Annu Rev Microbiol 2008 62:35-51.
- Blankschien MD, Potrykus K, Grace E, Choudhary A, Vinella D, Cashel M, Herman C. TraR, a homolog of a RNAP secondary channel interactor, modulates transcription. PLoS Genetics 2009 5:e10003345.
Collaborators
- Christophe Herman, PhD, Baylor College of Medicine, Houston, TX
- Agnieszka Szalewska-Palasz, PhD, University of Gdansk, Gdansk, Poland
- Daniel Vinella, PhD, Centre National de la Recherche Scientifique, Marseille, France
Contact
For more information, email cashel@mail.nih.gov or visit smr.nichd.nih.gov.