You are here: Home > Section on Developmental Biology
Molecular Genetics of Embryogenesis in Xenopus and Zebrafish
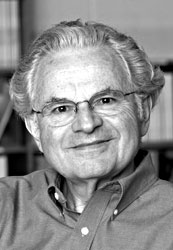
- Igor B. Dawid, PhD, Head, Section on Developmental Biology
- Emil Aamar, PhD, Visiting Fellow
- Sunit Dutta, PhD, Visiting Fellow
- John Gonzales, MA, MS, Technician
- Mi Ha Kim, PhD, Visiting Fellow
- Martha Rebbert, BS, Senior Technician
- Hyunju Ro, PhD, Research Fellow
- Reiko Toyama, PhD, Staff Scientist
- Valeria Zarelli, PhD, Visiting Fellow
The laboratory uses the frog Xenopus laevis and the zebrafish Danio rerio as experimental systems to study molecular-genetic mechanisms of early vertebrate development. Recently, we focused on gene discovery by DNA microarray technology and expression profiling in order to identify genes that play a role in the regulation of embryonic development. These approaches have led to studies on mechanisms of gastrulation, neural crest specification, and other patterning processes during embryogenesis.
Organizer restriction through modulation of Bozozok stability by the E3 ubiquitin ligase Lnx-like
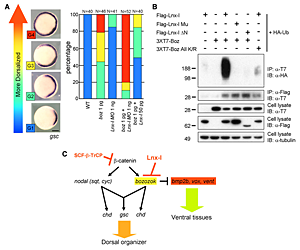
Click image to enlarge.
Figure 1. Lnx-like regulates organizer formation by controlling Boz stability
(A) Lnx-like counteracts Boz-mediated dorsalization, as measured by enhanced goosecoid (gsc) expression. Injected RNA or MO are shown beneath each bar. Lnx morpholino enhances and Lnx RNA suppresses Boz-induced dorsalization. (B) Lnx-like co-transfection into cultured cells leads to polyubiquitination and destabilization of Boz. Only wild-type but not mutant Box or Lnx-like are effective. (C) Model for regulatory interactions in early zebrafish embryos, leading to organizer formation. Lnx-like limits Boz accumulation and consequently the organizer domain.
In the vertebrate embryo, the organizer anchors the primary embryonic axis, and balance between dorsal (organizer) and ventral domains is fundamental to body patterning. We have studied a mechanism that regulates the size of the organizer domain by modulating the stability of a key molecule involved in organizer formation. This regulatory pathway is mediated by a member of the Ligand of Numb protein-X (Lnx) family, a RING finger– and four PDZ domain–containing E3 ubiquitin ligase. LNX proteins have been shown to serve as binding platforms and may have a role in cell fate determination, but their in vivo functions were previously unknown. We showed that Lnx-like, a novel member of the family in zebrafish, acts as a critical regulator of dorso-ventral axis formation in this animal. Depletion of Lnx-like using specific antisense morpholinos (MO) caused strong embryonic dorsalization (Figure 1A). We identified Bozozok (Boz; also called Dharma or Nieuwkoid) as a binding partner and substrate of Lnx-like (Figure 1B). Boz is a homeodomain-containing transcriptional repressor induced by canonical Wnt signaling that is critical for the formation of the dorsal organizer. Lnx-like induced lysine-48–linked polyubiquitination of Boz, leading to its proteasomal degradation both in human 293T cells and in zebrafish embryos. Dorsalization induced by Boz overexpression was suppressed by raising the level of Lnx-like, but Lnx-like failed to counteract dorsalization caused by a mutant of Boz that lacks a critical motif for Lnx-like binding. Further, dorsalization induced by depletion of Lnx-like was alleviated by attenuation of Boz expression. We conclude that Lnx-like modulates Boz activity to prevent the invasion of ventral regions of the embryo by organizer tissue. These studies introduce a ubiquitin ligase, Lnx-like, as a balancing modulator of axial patterning in the zebrafish embryo (Figure 1C). Further, we emphasize the fact that post-translational regulation of protein abundance mediated by the ubiquitin system has an important role in the establishment of the dorsal-ventral axis during early embryogenesis.
Coordinated activation of the secretory pathway during notochord formation in the Xenopus embryo
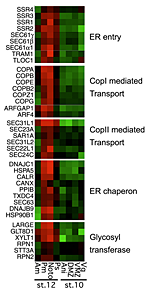
Click image to enlarge.
Figure 2. Secretory pathway genes in notochord development
Secretory pathway genes are enriched (red) in the notochord (noto) and the posterior mesoderm (pm) that includes the notochord in this DNA microarray–based analysis. No enrichment is seen in notochord precursors at earlier stages of development.
The notochord is the characteristic organ that gives the phylum chordates its name. Notochord development requires protein trafficking and secretion to form the sheath surrounding the tissue and the vacuoles within its cells. In a DNA microarray study, we found that activation of a large battery of genes encoding secretory pathway components is a characteristic feature of notochord differentiation in Xenopus (Figure 2). This coordinated activation is, at least in part, orchestrated by the activity of two transcription factors that also function in the unfolded protein response, where cells react to stress in their endoplasmic reticulum. We suggest that notochord differentiation involves a process similar to the unfolded protein response.
Neural crest formation in Xenopus and zebrafish embryos
We have studied neural crest specification since 1997, when this laboratory discovered the role of Wnt signaling in this process. Many signaling and transcription factors important in neural crest development have been identified in many laboratories in recent years. Nevertheless, novel components involved in the process are recognized regularly, and we have studied three such factors in the recent past.
Lrig3, a member of the leucine-rich repeats and immunoglobulin-like domains family, is expressed in the entire neural plate during neurulation and becomes localized in the developing neural crest during tailbud stages. Knockdown of Lrig3 by an antisense morpholino (MO) impairs neural crest formation, as visualized by several marker genes. Likewise, in the neural crest induction assay involving Chordin plus Wnt3a–injected animal caps, Lrig3 MO inhibited expression of the same markers. Lrig3 could attenuate Fgf signaling in animal caps, interacted with Fgf receptor 1 in cultured cells, and, according to context, reduced or augmented the induction of neural crest markers by Fgf. It is known that neural crest induction requires a precisely regulated level of Fgf signaling, and we suggest that Lrig3 functions by modulating the Fgf signaling pathway so as to keep its activity in the optimal range for neural crest formation.
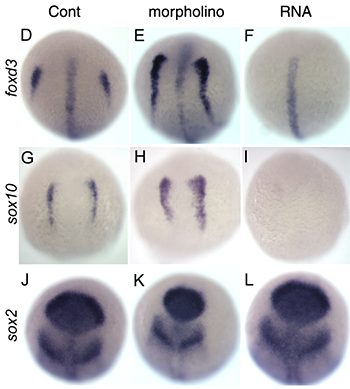
Click image to enlarge.
Figure 3. Regulation of neural crest formation in zebrafish
Manipulation of inhibitory factor expression affects neural crest development in zebrafish. The neural crest markers foxd3 and sox10, but not neural marker sox2, are increased by morpholino injection and inhibited by RNA injection. Note that foxd3 expression at the midline is unaffected while the neural crest expression in eliminated.
Among Myc family genes, c-Myc is known to play a role in neural crest specification in Xenopus and in craniofacial development in the mouse. However, no information was available on the function of other Myc genes in neural crest development or any developmental role of zebrafish Myc genes. We isolated the zebrafish mych (myc homolog) gene. Knockdown of mych leads to severe defects in craniofacial development and in the developmental of certain other tissues, including the eye. These phenotypes appear to be caused by cell death in the neural crest and in the eye field in the anterior brain. Thus, Mych is a novel factor required for neural crest cell survival in zebrafish.
More recently, we have studied a novel factor that appears to restrict neural crest induction. This neural crest–inhibitory factor appears to act downstream of the Wnt signaling pathway. Overexpression of the inhibitory factor blocks the expression of neural crest marker genes whereas injection of a MO against this factor leads to expansion of the neural crest domain, without substantial effect on the expression of neural markers (Figure 3). Further studies are directed at the mechanism of action of this novel factor.
FGF-dependent left-right asymmetry patterning in zebrafish is mediated by Ier2 and Fibp1
Establishment of left-right asymmetry in vertebrates has been studied extensively in recent years. This process has been shown to require nodal, Wnt-PCP, and Fgf signaling and involves ciliogenesis in a laterality organ such as the node in the mouse and Kupffer’s vesicle in zebrafish. While the involvement of FGF signaling in the process is known, effector genes through which Fgf affects laterality have not been described previously. We isolated the zebrafish ier2 and fibp1 genes as Fgf target genes and show that their protein products can interact with each other and co-localize to the nucleus. Knockdown of these factors with MOs interferes with establishment of organ laterality and with the asymmetric expression of marker genes such as the nodal gene southpaw. Ier2 and Fibp1 knock down causes defective cilia formation in Kupffer’s vesicle, likely explaining the defects in laterality establishment that arise subsequently. Cilia in Kupffer’s vesicle are also lost after suppression of Fgf8, but can be rescued by injection of ier2 and fibp1 mRNA (Figure 4). On the basis of these observations we conclude that Ier2 and Fibp1 mediate Fgf signaling in ciliogenesis in Kupffer’s vesicle and in the establishment of laterality in the zebrafish embryo.
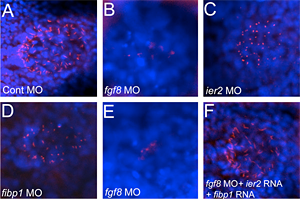
Click image to enlarge.
Figure 4. Cilia formation in Kupffer’s vesicle depends on Ier2 and Fibp1
Monocilia were detected with anti-acetylated tubulin antibody (red), and nuclei with DAPI (blue) in Kupffer’s vesicle of embryos injected with the indicated morpholinos. Morpholinos against fgf8 or ier2 and fibp1 lead to a drastic reduction of cilia, but ciliogenesis can be rescued by supplying ier2 and fibp1 RNA to fgf8 morpholino–injected embryos (F).
Pineal-specific gene expression in zebrafish
In a long-term collaboration with David Klein of this Institute and others, we carried out a DNA microarray–based analysis of the transcriptome in the zebrafish pineal gland at various stages of development. The data support the known role of the fish pineal gland as a photoreceptor organ, as genes involved in photoreception and transduction were prominent among the pineal-enriched genes. Developmental changes suggest that the embryonic pineal gland is enriched for regulatory factors such as transcription factors and signal transducers, whereas adult pineal glands express genes involved in physiological functions such as enzymes, photo-transduction factors, and structural components. The data have been deposited in public data bases and will be helpful in future studies of the regulation of pineal gland development and function.
Publications
- Zhao H, Tanegashima K, Ro H, Dawid IB. Lrig3 regulates neural crest formation in Xenopus by modulating Fgf and Wnt signaling pathways. Development 2008 135:1283-1293.
- Ro H, Dawid IB. Organizer restriction through modulation of Bozozok stability by the E3 ubiquitin ligase Lnx-like. Nat Cell Biol 2009 11:1121-1127.
- Tanegashima K, Zhao H, Rebbert ML, Dawid IB. Coordinated activation of the secretory pathway during notochord formation in the Xenopus embryo. Development 2009 136:3543-3548.
- Toyama R, Chen X, Jhawar N, Aamar E, Epstein J, Reany N, Alon S, Gothilf Y, Klein DC, Dawid IB. Transcriptome analysis of the zebrafish pineal gland. Dev Dyn 2009 238:1813-1826.
- Hong SK, Dawid IB. FGF-dependent left-right asymmetry patterning in zebrafish is mediated by Ier2 and Fibp1. Proc Natl Acad Sci USA 2009 106:2230-2235.
Collaborators
- Jonathan Epstein, MS, Scientific Software Support and Bioinformatics Core Facility, NICHD, Bethesda, MD
- Yoav Gothilf, PhD, Tel Aviv University, Tel Aviv, Israel
- David Klein, PhD, Program on Developmental Endocrinology and Genetics, NICHD, Bethesda, MD
- Michael Tsang, PhD, University of Pittsburgh, Pittsburgh, PA
Contact
For more information, email dawidi@mail.nih.gov or visit sdb.nichd.nih.gov.