You are here: Home > Section on Viral Gene Regulation
Molecular Genetics of Mammalian Retrovirus Replication
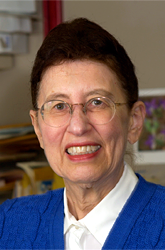
- Judith G. Levin, PhD, Head, Section on Viral Gene Regulation
- Amber L. Hertz, BS, Postbaccalaureate Fellow
- Kamil Hercik, PhD, Postdoctoral Fellow
- Christopher B. Hergott, BS, Postbaccalaureate Fellow
- Jiyang Jiang, MD, PhD, Postdoctoral Fellow
- Mithun Mitra, PhD, Postdoctoral Fellow
- Klara Post, MS, Senior Research Assistant
- Tiyun Wu, PhD, Staff Scientist
The goal of the research performed in the Section on Viral Gene Regulation is to define the molecular mechanisms responsible for the replication of HIV and related retroviruses and to investigate the role of host proteins that block virus infection. These studies are critical for developing new strategies to combat the AIDS epidemic, which continues to be a global threat to human health. To address this issue, we have developed reconstituted model systems to investigate the individual steps in HIV-1 reverse transcription, a major target of HIV therapy. Much of our work focuses on the viral nucleocapsid protein (NC), a nucleic acid chaperone facilitating nucleic acid conformational rearrangements that lead to formation of the most thermodynamically stable structures. This activity is critical for highly efficient and specific viral DNA synthesis. We are also studying the mechanism of antiviral activity of two host cytidine deaminases: human APOBEC3G (A3G) and APOBEC3A (A3A). In other studies, our efforts are directed toward understanding the function of the viral capsid protein (CA) in HIV-1 assembly and early postentry events during the course of virus replication in vivo.
Role of nucleocapsid protein in HIV-1 reverse transcription
HIV-1 NC is a small basic protein with two zinc fingers, each containing the invariant CCHC zinc-coordinating residues. Its nucleic acid chaperone function consists of two independent activities: nucleic acid aggregation, which is important for annealing (N-terminal basic residues); and weak helix-destabilizing activity (zinc fingers). NC plays a critical role in almost every step of viral DNA synthesis, including minus-strand transfer. In this case, the first product of reverse transcription, (−) strong-stop DNA, is annealed to the RNA sequence at the 3′ end of the genome (acceptor RNA), in a reaction facilitated by base-pairing of the complementary repeat regions in the nucleic acid substrates. This is followed by reverse transcriptase– (RT) catalyzed elongation of minus-strand DNA. Current studies focus on a comparison of the nucleic acid chaperone activities of HIV-1 NC with HIV-1 Gag and its proteolytic cleavage products. The sequence of Gag in the N→C direction is: matrix (MA)→capsid (CA)→spacer peptide 1 (SP1)→NC→SP2→p6. For this work, we use a reconstituted minus-strand transfer assay system, an especially sensitive read-out for chaperone function, given that both strand annealing and duplex destabilization are required for a positive result.
We found for the first time that Gag facilitates minus-strand transfer, although, at optimal concentrations of Gag and NC, Gag activity was about 1.5-fold lower than that of NC. In annealing-only assays, high concentrations of Gag stimulated annealing more strongly than did NC. This result presumably reflects Gag’s strong nucleic acid–binding activity (approximate Kd values: Gag, 9 nM; NC, 108 nM). In contrast, high concentrations of Gag severely inhibited the DNA extension step, whereas NC did not. This behavior is likely attributable to the known slow dissociation rate of Gag bound to single-strand nucleic acids, which could lead to inhibition of DNA elongation by a “roadblock” mechanism. Gag proteins lacking all or part of MA, but having the NC domain, retained the ability to stimulate minus-strand transfer; however, Gag proteins lacking the NC domain (MA, MACA, or CA) exhibited no chaperone activity. To evaluate Gag function in a viral setting, we also examined the endogenous RT (ERT) activity of virions expressing Gag or partially processed Gag proteins generated by blocking protease cleavage sites (mutants from C. Aiken, Vanderbilt University). In collaborative studies, real-time PCR analysis of ERT reactions showed that constructs able to release mature NC could undergo minus-strand transfer as efficiently as wild type. Virions expressing uncleaved Gag or a partially cleaved Gag (MA-CA-SP1-NC) had about 29% and 55% of wild-type activity, respectively. These findings are in accord with our in vitro experiments. Taken together, our results demonstrate that the critical determinant for the nucleic acid chaperone activity of Gag resides in its NC domain. Moreover, the ability of high concentrations of Gag to act as a roadblock to DNA elongation represents a novel regulatory mechanism to prevent premature reverse transcription.
In other work, recent studies on HIV-1 plus-strand DNA initiation by the 3′ RNA polypurine tract (PPT) primer have led to the discovery of a previously unrecognized function for NC nucleic acid chaperone activity: blocking mispriming by non-PPT RNAs. These RNAs are generated during RNase H degradation of viral RNA during minus-strand DNA synthesis and are potential plus-strand primers. If mispriming were to occur, viral DNA would not have the correct ends and would not be competent for integration. Our approach was to test three purine-rich 20-nt RNAs from the PPT region in primer extension assays. NC reduced priming by these RNAs to an essentially base-line level, whereas PPT priming was unaffected. RNase H cleavage and NC destabilization of non-PPT duplexes, an activity associated with intact zinc fingers, were required for maximal inhibition of mispriming. Collaborative experiments measuring biophysical properties of short duplexes showed significant differences between PPT and non-PPT duplexes, in accord with the biochemical data. Collectively, these results demonstrate that NC chaperone activity plays a major role in ensuring the fidelity of plus-strand priming. We propose that the mechanism for NC’s activity is based on its ability to destabilize nucleic acid duplexes that have a moderately stable secondary structure. In turn, this also explains why the unique structure of the PPT renders it resistant to NC. Experiments are now in progress to investigate the effects of NC precursors on mispriming.
Molecular analysis of the functional activities of human APOBEC proteins
Our interest in host proteins that might affect HIV-1 reverse transcription led us to investigate human A3G, a cellular cytidine deaminase with two zinc-finger domains, which blocks HIV-1 reverse transcription and replication in the absence of the viral protein known as Vif. The antiviral effect has been shown to be largely deaminase-dependent, but there is also a deaminase-independent component. Previously, we succeeded in purifying catalytically active A3G expressed in a baculovirus system, which allowed us to provide a comprehensive molecular analysis of A3G’s deaminase and nucleic acid–binding activities. A major focus of our A3G studies has been to elucidate the mechanism for A3G inhibition of reverse transcription. Using purified proteins, we investigated the interplay between A3G, NC, and RT in reconstituted reactions representing individual steps in the reverse transcription pathway. We reported that A3G does not affect the kinetics of NC-mediated annealing or the RNase H activity of RT. In sharp contrast, A3G significantly inhibits all RT–catalyzed elongation reactions with or without NC and without a requirement for A3G catalytic activity. Data from single-molecule DNA stretching analyses and fluorescence anisotropy support an unusual roadblock mechanism for deaminase-independent inhibition of reverse transcription that is determined by critical differences in the nucleic acid binding properties of A3G, NC, and RT. It is interesting that both A3G and Gag function as roadblocks to DNA extension, although the effect of the inhibition differs in each case. This suggests that we have uncovered a more general mechanism for modulating viral DNA synthesis.
In current work, we have begun to study the human APOBEC3A (A3A) protein, a cytidine deaminase that has only one zinc-finger domain and is a potent inhibitor of retrotransposition by Line-1 and Alu non–LTR elements. We expressed A3A with a GST tag in E. coli and purified the protein from bacterial extracts. Efforts are under way to find conditions that will yield larger amounts of the purified untagged protein for detailed biochemical and structural analysis. An assay to measure Line-1 retrotransposition is being developed, and construction of structure-guided A3A mutations for use in this assay is in progress. Interestingly, a model of A3A structure indicates that it is similar to the X-ray crystal structure of the C-terminal domain of A3G. Indeed, one of our collaborators has shown that a chimeric protein with the N-terminal domain of A3G linked to A3A inhibits HIV-1 replication. We are now investigating the properties of the chimera containing either wild-type or mutant A3A sequences.
Function of HIV-1 capsid protein in virus assembly and early post-entry events
Our laboratory has been investigating the role of the HIV-1 capsid protein (CA) in early postentry events, a stage in the infectious process that is still not completely understood. In our initial study, we described the unusual phenotype associated with single alanine substitution mutations in a group of N-terminal, conserved hydrophobic residues (including Trp23 and Phe40), using genetic, molecular, and ultrastructural approaches. We found that mutant virions are not infectious, lack a cone-shaped core, and despite having a functional RT enzyme, are blocked in the initiation of viral DNA synthesis in infected cells. Mutant viral cores display a marked deficiency in RT and have substantially increased amounts of CA, suggesting a defect in core disassembly. Taken together, these findings illuminate the intimate connection between infectivity, proper core morphology, structural integrity of the CA protein, and ability to undergo reverse transcription.
To pursue these findings in more detail, we performed a study to provide new information on the plasticity of CA, i.e., its ability to tolerate changes in residues crucial for CA structure without total abrogation of biological activity. Mutants were constructed and tested to determine whether they might retain the ability to replicate and thereby present an opportunity to isolate second-site suppressors. One mutant, W23F, was found to exhibit infectivity in a single-cycle assay, albeit at a very low level. W23F has a phenotype that is intermediate between wild-type virus and the original W23A mutant. The W23F mutant (unlike W23A) is able to replicate during long-term culture in MT-4 cells, but with delayed replication kinetics. With continued passage, we could eventually isolate virions with a second-site suppressor mutation (W23F/V26I). The suppressor mutation partially restores the wild-type phenotype, including production of particles with conical cores and normal CA content, as well as wild-type replication kinetics in MT-4 cells. A structural model that accommodates the spatial changes induced by the W23F and V26I mutations shows that hydrophobic interactions between Phe23 and Ile26 are possible, explaining the suppressor phenotype. These findings are novel and demonstrate that despite the spatial constraints imposed on assembly of CA structure, HIV-1 is able to adapt, at least partially, to severe structural distortions in a major viral protein.
With advances in X-ray crystallography and the development of new electron microscopic techniques, such as cryo-electron tomography, there have been a number of reports on the three-dimensional structure of the hexameric arrays of full-length HIV-1 CA. Nevertheless, there is still little information on the nature of the short interdomain linker peptide (residues 146-150; SPTSI) that connects the N- and C-terminal domains. In current work, we have made alanine-scanning mutations in all residues in the linker region as well as in the two residues (Y145 and L151) that flank the linker on either side. Except for P147A, all these mutants produce virus particles; we have therefore substituted P147L in this series. The mutants also have the normal complement of viral proteins. However, of the five linker mutants, only two (S146A and T148A) have substantial infectivity in a single-cycle replication assay; Y145A and L151A are not infectious. The lack of infectivity is correlated with the appearance of aberrant cores, as observed by transmission electron microscopy, detergent sensitivity and instability of the cores, and significantly reduced ability to make viral DNA in infected cells as well as dominant-negative inhibition of wild-type infectivity. These findings demonstrate that the linker peptide is a critical element in determining proper HIV-1 CA structure. Further characterization of these mutants is in progress.
Publications
- Post K, Kankia B, Gopalakrishnan S, Yang V, Cramer E, Saladores P, Gorelick RJ, Guo J, Musier-Forsyth K, Levin JG. Fidelity of plus-strand priming requires the nucleic acid chaperone activity of HIV-1 nucleocapsid protein. Nucleic Acids Res 2009 37:1755-1766.
Collaborators
- Eric O. Freed, PhD, HIV Drug Resistance Program, NCI at Frederick, Frederick, MD
- Robert J. Gorelick, PhD, AIDS and Cancer Virus Program, SAIC-Frederick, Inc., NCI at Frederick, Frederick, MD
- Angela M. Gronenborn, PhD, University of Pittsburgh Medical School, Pittsburgh, PA
- Karin Musier-Forsyth, PhD, Ohio State University, Columbus, OH
- Alan Rein, PhD, HIV Drug Resistance Program, NCI at Frederick, Frederick, MD
- Ioulia Rouzina, PhD, University of Minnesota, Minneapolis, MN
- Klaus Strebel, PhD, Laboratory of Molecular Microbiology, NIAID, Bethesda, MD
- Shixing Tang, MD, PhD, Center for Biologics Evaluation and Research, FDA, Bethesda, MD
- Mark C. Williams, PhD, Northeastern University, Boston, MA
- Yasumasa Iwatani, PhD, National Hospital Organization Nagoya Medical Center, Nagoya, Japan
Contact
For more information, email levinju@mail.nih.gov or visit jlevinlab.nichd.nih.gov.