You are here: Home > Section on Molecular Regulation
Biologically Ubiquitous Global Regulation of Gene Expression by ppGpp
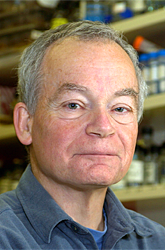
- Michael Cashel, MD, PhD, Head, Section on Molecular Regulation
- Katarzyna Potrykus, PhD, Research Fellow
- Daniel Vinella, PhD, Research Scholar
- Agnieszka Szalewska-Palasz, PhD, Research Scholar
The pppGpp and ppGpp nucleotides are two analogs that are related to GTP and GDP but whose ribose 3' hydroxyl group is esterified with pyrophosphate residues. Collectively, they are abbreviated here as (p)ppGpp. In our in previous reports, we described these nucleotides as regulators of global gene expression, but only in bacteria and plants. The (p)ppGpp nucleotides have been most extensively studied in bacteria, in which they are known to act as second messengers to signal stress that provokes either positive or negative regulation of global gene expression at the transcriptional level and to ultimately control growth and adaptation to stress. In apparent defiance of the usual rules of regulatory specificity, bacteria can use (p)ppGpp to signal limitation in almost any nutrient (nitrogen, carbon, amino acids, some vitamins, phosphate, iron, or oxygen) as well some physical sources of stress (membrane, pH, osmotic, or heat). General features of these responses are reviewed in reference 2.
This year, another laboratory reported that (p)ppGpp and its regulatory effects on gene expression are very likely to be biologically ubiquitous (Sun et al., Nat Struct Biol. Sept. 2010 advance online publication). This is because worms, fruit flies, and humans have been found to possess a crucial enzyme (Mesh1) that specifically catalyzes (p)ppGpp hydrolysis. The structure of the catalytic sites of the D. melanogaster and human enzymes is essentially identical to its bacterial counterpart. The deduction that (p)ppGpp has global regulatory effects is based on the following observations: 1) deletion of Mesh1 is expected to elevate (p)ppGpp levels, although as yet this nucleotide has yet to be detected; 2) the Mesh1 deletion provokes regulatory global gene expression effects on major cellular processes, as occur in bacterial with excess (p)ppGpp; 3) in fruit flies, overexpression of a bacterial (p)ppGpp synthetase demonstrably elevates (p)ppGpp, resulting in elevated sensitivity to nutrient starvation and several developmental stage-specific anomalies (we have deduced that deletion of Mesh1, which encodes (p)ppGpp hydrolase, elevates (p)ppGpp because, in the same organism, it also confers starvation sensitivity and similar developmental anomalies); 4) overexpression of Mesh1 complements phenotypic defects generated by the Mesh1 deletion noted in 2).
As noted in earlier reports, bacterial (p)ppGpp is frequently important for pathogenesis, production of toxins, formation of biofilms, elaboration of pheromones, and expression of virulence genes that arise from horizontal transfer between species. When Gram-negative pathogens are made (p)ppGpp-deficient, pathogenicity is compromised or lost completely. The literature is incomplete for Gram-positives, in which ppGpp-deficient artificial constructs are rare due to redundant sources for (p)ppGpp synthesis. Accordingly, we have extended the goal of our research to understand the regulation of gene expression in bacteria at the level of transcription as well as to explore role of (p)ppGpp in animal cells, focusing on transcription and regulation by (p)ppGpp. In our opinion, current evidence suggests that (p)ppGpp represents a regulatory signal conserved throughout biology.
Discovery of a cluster of ll RNAs, called GraLsma
The GraL cluster arises in the greA leader transcript initiated by two promoters: a house-keeping (sigma-70) promoter and a ppGpp-dependent stress (sigma-E) promoter. The GreA protein has long been known to function in transcription by restarting RNA polymerase transcripts that have become arrested during elongation when a growing RNA chain slips off of the DNA template within the enzyme such that the growing (3'OH end) of RNA is no longer positioned within the catalytic site. GreA is thought to relieve elongation arrest through a conformational change in RNA polymerase that activates an otherwise silent RNase; this cleavage of the slipped RNA, in turn, restores alignment of the 3'OH end of nascent RNA with the catalytic site to allow continued elongation. Our previous annual reports described our discovery that GreA has yet other functions: it can activate transcription initiation of a ppGpp-regulated promoter that drives ribosomal RNA synthesis and also reverse several phenotypes caused by the absence of (p)ppGpp.
The leader termination event is of particular interest because it gives rise to a highly unusual population of small RNA chains that we collectively call GraL. The GraL cluster contains terminated transcripts whose ends differ in length by up to 12 nt. This extent of imprecise termination has not been observed before for bacterial transcription termination mediated by RNA polymerase alone; rather, the lengths of terminated RNA chains usually differ by no more than a few nucleotides. Because of the novelty of GraL, we made a major effort to characterize the termination phenomenon. We documented that the GraL transcript chain lengths are variable because they have different 3′OH ends rather than start sites, because two RNA chain clusters are formed in vitro with an interval between the clusters equal to the distance between the two promoters. We used immobilized transcription complexes to show that the GraL RNA chains are physically released from RNA polymerase during termination. This excludes the possibility that the GraL size distribution is due to transcription arrest. Genetic evidence with RNase gene deletions indicates that the frayed ends are not due to ribonuclease cleavage or processing but rather reflect actual termination sites; this can be demonstrated in vitro with an array of antisense oligonucleotides to various portions of the abnormally long terminator stem-loop. The studies also define the lower portions of the stem loop as determinants of incremental termination. Given that the frayed ends are visualized by Northern blots with RNA preparations from growing cells, GraL is not an in vitro artifact.
Surprisingly, the abundance of GraL itself does not seem to be regulated; if it were, an increase in GraL abundance would be associated with diminished GreA expression. Therefore the existence of GraL does not change cellular greA expression, raising the question of why GraL is present. Sequence comparisons among closely related bacteria reveal sequence divergence in the GraL region, yet RNA folding reveals that the terminator structures are conserved, evidence that favors evolutionary conservation of the GraL termination function. We investigated the biological importance of GraL by measuring a difference in fitness or selective advantage of GraL-overexpressing cells vs. cells not overexpressing GraL during repeated cycles of overnight growth in LB media. This was done by inoculating cultures with equal admixtures of cells containing GraL-overproducing plasmids (or vector) with either one or the other marked with a malT mutant. The proportion of maltose-fermenting cells at any time of culture could be scored as the ratio of red and white colonies on maltose indicator plates. Cells overexpressing GraL were found to reproducibly and systematically out-compete wild-type cells whether or not the malT marker mutation was present together with the GraL plasmid. A scrambled GraL sequence control did not similarly affect fitness.
We also performed microarray analyses to search for target genes whose transcription is influenced by GraL. When GraL was overproduced for 15 minutes, no effect was found in wild-type strains. However, in the absence of ppGpp, over 100 genes were affected. Specifically, many genes affected by GraL are known to be repressed by Fur, a major regulator of iron metabolism. This is to be expected, given that, many years ago, we found that ppGpp responds to iron starvation. Flagellar genes also constitute a general class that is inhibited by GraL. Again, studies in our laboratory and those of others have implicated ppGpp (and a synergistic protein, DksA) in the regulation of flagellar genes. Computer searches that look for RNA-RNA hybrids as mediators of small RNA regulation did not identify mRNA targets that could be verified in the microarray results or by direct testing. Genetic evidence also excluded RNaseIII and Hfq as involved in regulation by this small RNA. The results of this work indicate that GraL arises from a highly unusual series of termination events and that GraL has a biologically significant function. However, specific targets and mechanisms of action for these targets have not yet been identified.
ppGpp is the major source of growth rate control in E. coli.
This year, we re-evaluated the role of ppGpp as a determinant of variation of the E. coli cellular content of DNA, RNA, and protein as a function of growth rate (reference 5). It has been known for over 50 years that the cellular content of these macromolecules is controlled solely by the exponential growth rate and not by the composition of the growth media needed to achieve a given growth rate. This counter-intuitive phenomenon is called growth rate control. There is also a consensus that growth rate control is attributable to variation of the number of ribosomes per cell that all function at near optimal efficiency for protein synthesis; i.e., faster growing cells contain more ribosomes, enabling them to make more protein to grow faster. Cellular DNA content shows only a modest increase with increasing growth rate, relative to RNA content. The possible alternative mechanism is that the number of ribosomes per cell is fixed but that the efficiency of each ribosome for protein synthesis varies with growth rate. It is well known that ppGpp negatively regulates rRNA synthesis at the level of transcription during the stress of amino acid starvation but, until our recent work, uncertainty persisted as to whether ppGpp causes growth rate control at all, in part, or fully. The straightforward approach to answer this question is to determine whether growth rate control still occurs in cells completely lacking (p)ppGpp. We constructed such strains about 20 years ago, and these constructs were used by several labs to report disparate conclusions over the past 10 years. Using similar strains, our re-evaluation differs from earlier experiments through the use of sensitive RNA-specific and DNA-specific fluorescent assay techniques (fluorescent dyes) for measuring RNA and DNA, development of nutritionally defined media for growing ppGpp-deficient cells, and special culture conditions to avoid suppressor mutations in RNA polymerase that otherwise confound results because the mutants themselves alter growth rate control (see reference 3).
We found that when (p)ppGpp is absent, cellular RNA/DNA and RNA/protein ratios are as high as in fast-growing wild-type cells, which contain low basal levels of (p)ppGpp. In contrast, slow-growing wild-type cells have high (p)ppGpp basal levels and low RNA/DNA and RNA/protein ratios as a result of fewer ribosomes per cell. Also, artificial elevation of (p)ppGpp or introduction of RNA polymerase mutants (mentioned above) restores growth rate control. Finally, characterization of ribosomal particles themselves and their subunits present under various growth conditions reveal that the large number of mature ribosomes formed at slow growth rates in the absence of (p)ppGpp appear normal. Thus, either all overproduced ribosomes function at low efficiency or a tiny fraction functions at high efficiency. In any event, the absence of (p)ppGpp constitutes the first instance of overexpression of ribosomes under physiological conditions of slow balanced growth. We believe our findings finally resolve this long-standing controversy, given the strong evidence that (p)ppGpp is the major factor responsible for growth rate control in E. coli, and offer a plausible explanation for earlier conflicting observations. This finding greatly simplifies our understanding of the basic physiological changes during growth of E. coli.
Publications
- Potrykus K, Murphy H, Chen X, Epstein JA, Cashel M. Imprecise transcription termination within Escherichia coli greA leader gives rise to an array of short transcripts, GraL. Nucleic Acids Res. 2010; 38:1636-51.
- Szalewska-Palasz A, Potrykus K, Cashel M, Wegrzyn G. Starvation of bacteria for amino acids as an example of prokaryotic response to nutritional deprivation. The Biology of Starvation in Humans and Other Organisms 2010; in press.
- Potrykus K, Cashel M,. Stringent Mutant / Stringent Response. Brenner's Online Encyclopedia of Genetics. 2010; in press.
- James T, Cashel M, Hinton D. A mutation within the beta subunit of Escherichia coli RNA polymerase impairs transcription from bacteriophage T4 middle promoters. J Bacteriol. 2010; in press.
- Potrykus K, Murphy M, Philippe N, Cashel M. ppGpp is the major source of growth rate control in E. coli. Environ Microbiol. 2010; in press.
Collaborators
- Christophe Herman, PhD, Baylor College of Medicine, Houston, TX
- Agnieszka Szalewska-Palasz, PhD, University of Gdansk, Gdansk, Poland
- Daniel Vinella, PhD, Centre National de la Recherche Scientifique, Marseille, France
Contact
For more information, email cashel@mail.nih.gov or visit smr.nichd.nih.gov.