You are here: Home > Section on Viral Gene Regulation
Molecular Genetics of Mammalian Retrovirus Replication
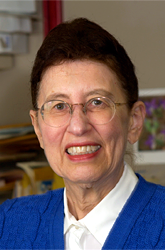
- Judith G. Levin, PhD, Head, Section on Viral Gene Regulation
- Tiyun Wu, PhD, Staff Scientist
- Kamil Hercik, PhD, Postdoctoral Fellow
- Jiyang Jiang, MD, PhD, Postdoctoral Fellow
- Mithun Mitra, PhD, Postdoctoral Fellow
- Klara Post, MS, Senior Research Assistant
- Christopher B. Hergott, BS, Postbaccalaureate Fellow
- Kathryn S. Hinchee, BS, Postbaccalaureate Fellow
The goal of the research performed in the Section on Viral Gene Regulation is to define the molecular mechanisms responsible for the replication of HIV and related retroviruses and to investigate the role of host proteins that block virus infection. The studies are critical for developing new strategies to combat the AIDS epidemic, which continues to be a global threat to human health. To address this issue, we have developed reconstituted model systems to investigate the individual steps in HIV-1 reverse transcription, a major target of HIV therapy. Much of our work focuses on the viral nucleocapsid protein (NC), a nucleic acid chaperone that remodels nucleic acid structures so that the most thermodynamically stable conformations are formed. This activity is critical for highly efficient and specific viral DNA synthesis. We are also investigating the mechanism of antiviral activity of two human cytidine deaminases, APOBEC3G (A3G) and APOBEC3A (A3A). In other studies, our efforts are directed toward understanding the function of the viral capsid protein (CA) in HIV-1 assembly and early postentry events during the course of virus replication in vivo.
Role of nucleocapsid protein in HIV-1 reverse transcription
HIV-1 NC is a small basic protein bearing two zinc fingers, each containing the invariant CCHC zinc-coordinating residues. Its nucleic acid chaperone function depends on three properties: 1) ability to aggregate nucleic acids, which is important for annealing (N-terminal basic residues); 2) moderate helix destabilizing activity (zinc fingers); and 3) rapid on-off binding kinetics. NC plays a critical role in almost every step of viral DNA synthesis, including minus-strand transfer. In this case, the first product of reverse transcription, (−) strong-stop DNA, is annealed to the RNA sequence at the 3' end of the genome (acceptor RNA), in a reaction mediated by base-pairing of the complementary repeat regions in the nucleic acid substrates. This is followed by reverse transcriptase (RT)–catalyzed elongation of minus-strand DNA. Current studies focus on a comparison of the nucleic acid chaperone activities of HIV-1 NC with HIV-1 Gag and its proteolytic cleavage products. The sequence of Gag in the N to C direction is: matrix (MA)–capsid (CA)–spacer peptide 1 (SP1)–NC–SP2–p6. For this work, we use a reconstituted minus-strand transfer assay system, an especially sensitive read-out for chaperone function.
We were the first to find that Gag facilitates minus-strand transfer, indicating that it has both annealing and helix-destabilizing activities. However, at optimal concentrations of Gag and NC, Gag activity in minus-strand transfer is ~1.5-fold lower than that of NC. To compare the activities of Gag and NC in the individual steps of strand transfer, we performed assays of annealing only. The experiments showed that high concentrations of Gag stimulate annealing more strongly than NC, a result that reflects Gag's 15-fold higher affinity for binding to single-stranded (ss) RNA and ssDNA, as determined by fluorescence anisotropy. In contrast, high concentrations of Gag severely inhibit the DNA extension step. This was also seen, albeit to a lesser extent, with partially processed Gag proteins, but not with NC. The results are consistent with "nucleic acid–driven multimerization" of Gag (facilitated by CA domain interactions) and the known slow dissociation of Gag from bound nucleic acid, which in turn block RT movement along the ss nucleic acid template. We refer to this phenomenon as the "roadblock" mechanism for inhibition of HIV-1 reverse transcription. Gag-derived proteins that lack all or part of MA but have the NC domain retain the ability to stimulate minus-strand transfer; however, Gag-derived proteins lacking the NC domain (MA, MACA, or CA) have no chaperone activity. To evaluate Gag function in a viral setting, we also examined the endogenous RT (ERT) activity of virions expressing Gag or partially processed Gag proteins generated by blocking protease cleavage sites (mutants from C. Aiken, Vanderbilt University). Real-time PCR analysis of ERT reactions showed that constructs able to release mature NC could undergo minus-strand transfer as efficiently as wild type. Virions expressing uncleaved Gag or a partially cleaved Gag (MA-CA-SP1-NC) have ~29% and ~55% of wild-type activity, respectively. These findings are in accord with our in vitro experiments.
Taken together, the results demonstrate that the critical determinant for the nucleic acid chaperone activity of Gag resides in its NC domain. Moreover, the work illustrates how the presence of NC in the multi-domain Gag protein modulates the nature of its nucleic acid chaperone activity and also helps to explain why NC, rather than the Gag precursor, has evolved as the critical cofactor in viral DNA synthesis. Interestingly, the ability of high concentrations of Gag to act as a roadblock to DNA elongation represents a novel regulatory mechanism, which in principle, could inhibit premature reverse transcription.
In other work, our recent studies on HIV-1 plus-strand DNA initiation by the 3' RNA polypurine tract (PPT) primer led to the discovery of a previously unrecognized function for NC nucleic acid chaperone activity: blocking mispriming by non-PPT RNAs. These RNAs are generated during the RNase H degradation of viral RNA that occurs during minus-strand DNA synthesis and are potential plus-strand primers. If mispriming were to occur, viral DNA would not have the correct ends and would not be competent for integration. Our approach was to test three purine-rich 20-nt RNAs from the PPT region in primer extension assays. NC reduces priming by these RNAs to essentially base-line level, whereas PPT priming is unaffected. RNase H cleavage and NC destabilization of non-PPT duplexes, an activity associated with intact zinc fingers, are required for maximal inhibition of mispriming. Experiments measuring the biophysical properties of short duplexes showed significant differences between PPT and non-PPT duplexes, in accord with the biochemical data. Collectively, these results demonstrate that NC chaperone activity has a major role in ensuring the fidelity of plus-strand priming. We propose that the mechanism for NC's activity is based on its ability to destabilize nucleic acid duplexes with a moderately stable secondary structure. In addition, this also explains why the unique structure of the highly stable PPT renders it resistant to NC.
The immediate precursors of NC (sometimes referred to as NC7) are NC9 (NC + SP2) and NC15 (NC9 + p6). To provide further insights into the mechanism of nucleic acid chaperone function, it is of interest to compare the activities of these precursors to those of NC and Gag. In one approach, we are investigating the effects of these precursors on mispriming. In recent work, we found that, with a 20-nt duplex, the three NC proteins inhibit mispriming to approximately the same extent. Experiments are now in progress to investigate the biochemical and biophysical effects of NC and its precursors on larger duplexes. We are also studying the effects of the precursors on other NC-mediated steps in reverse transcription.
Molecular analysis of the functional activities of human APOBEC proteins
Our interest in host proteins that might affect HIV-1 reverse transcription led us to investigate human A3G, a cellular cytidine deaminase with two zinc finger domains, which blocks HIV-1 reverse transcription and replication in the absence of the viral protein known as Vif. The antiviral effect has been shown to be largely deaminase-dependent, but there is also a deaminase-independent component. Previously, we succeeded in producing highly purified, catalytically active A3G expressed in a baculovirus system. A major focus of our A3G studies has been to elucidate the mechanism for A3G inhibition of reverse transcription. Using purified proteins, we investigated the interplay between A3G, NC, and RT in reconstituted reactions representing individual steps in the reverse transcription pathway. We reported that A3G does not affect the kinetics of NC-mediated annealing or the RNase H activity of RT. In sharp contrast, A3G significantly inhibits all RT-catalyzed elongation reactions with or without NC and without a requirement for A3G catalytic activity. Our results support an unusual roadblock mechanism for deaminase-independent inhibition of reverse transcription that is determined by critical differences in the nucleic acid binding properties of A3G, NC, and RT. It is interesting that both A3G and Gag function as roadblocks to DNA extension. This suggests that we have uncovered a more general mechanism for modulating viral DNA synthesis.
Recently, we were involved in a collaborative study that used structure-guided mutagenesis of A3G to identify the four surface-exposed Lys residues (out of 14) that are required for Vif-mediated A3G ubiquitination and degradation via the proteasomal pathway. Substitution of Arg for these residues confers resistance to Vif and restores A3G's antiviral activity. In our model, the critical four Lys residues cluster at the C-terminus, opposite to the known N-terminal Vif interaction domain in A3G. Taken together, our results provide insights into the determinants governing Vif-mediated A3G inactivation and should stimulate and aid efforts to develop antiviral strategies that focus on disruption of the Vif-A3G connection.
In current work, we have been studying the human A3A protein, a cytidine deaminase that has only one zinc finger domain and is a potent inhibitor of retrotransposition by Line-1 and Alu non-LTR elements. We expressed A3A in E. coli and purified the protein from bacterial extracts. In collaboration with a structural biology group, which has the capacity to produce large quantities of expressed proteins, we recently obtained sufficient material for detailed biochemical and structural analysis. Preliminary NMR spectra show that purified A3A is well structured in solution, and experiments are now in progress to determine its three-dimensional structure. We are also investigating the requirements for A3A's cytidine deaminase activity and its binding properties. We found that A3A binds to nucleic acid much less efficiently than does A3G, presumably because A3A has only one zinc-binding domain and is an acidic protein. In addition, we are using structure-guided mutagenesis to identify residues of biological importance, as determined with a Line-1 retrotransposition assay. Interestingly, a model of A3A structure indicates that it is similar to the X-ray crystal structure of the C-terminal domain of A3G. Indeed, one of our collaborators has shown that a chimeric protein with the N-terminal domain of A3G linked to A3A inhibits HIV-1 replication. We are now investigating the properties of the chimera containing either wild-type or mutant A3A sequences.
Function of HIV-1 capsid protein in virus assembly and early post-entry events
Our laboratory has been investigating the role of the HIV-1 capsid protein (CA) in early postentry events, a stage in the infectious process that is still not completely understood. Our initial studies illuminated the intimate connection between infectivity, proper core assembly, structural integrity of the CA protein, and ability to undergo reverse transcription. In subsequent work, we isolated a second-site suppressor of a deleterious hydrophobic amino acid mutation, which partially restored the wild-type phenotype, including production of particles with conical cores, normal CA content, and normal replication kinetics. The findings are novel and demonstrate that, despite the spatial constraints imposed on assembly of CA structure, HIV-1 is able to adapt, at least partially, to severe structural distortions in a major viral protein.
With advances in X-ray crystallography and the development of new electron microscopic techniques, such as cryo-electron tomography, there have been a number of reports on the three-dimensional structure of the N-terminal hexameric arrays of full-length HIV-1 CA and their interaction with C-terminal dimers. Nevertheless, there is still little information on the nature of the short interdomain hinge or linker peptide (residues 146-150; SPTSI) that connects the N- and C-terminal domains. In current work, we have made alanine-scanning mutations in all residues in the hinge region (except for P147, which was changed to Leu), as well as in the two flanking residues (Y145 and L151). The mutants all produced particles, but only S146A and T148A had substantial infectivity. Transmission EM revealed that three of the five non-infectious mutants, Y145A, I150A, and L151A, had eccentric or centric cores, but no conical cores. Surprisingly, two other mutants in this group, P147L and S149A, had a mixed population of conical cores (~50% of the wild-type value) as well as aberrant cores. Similarly, in vitro assemblies performed with purified wild-type and mutant CA proteins showed that P147L and S149A formed a mixture of long tubes (like those formed by wild-type CA) and short tubes, whereas I150A did not assemble into any recognizable structure. The distinctive nature of the P147L and S149A mutants was also apparent in other assays. For example, when viral DNA products were assayed in infected cells by qPCR, both mutants synthesized about 10-fold less DNA than wild type. In strong contrast, I150A made ~104-fold less DNA than wild type. Taken together, our results clearly demonstrate that perturbation of the CA protein in the hinge region leads to distortion of core architecture, with downstream effects on reverse transcription and infectivity. The findings demonstrate that the hinge region is a critical element in determining proper HIV-1 CA structure. Further analysis of the P147L and S149A mutations is now in progress.
Publications
- Post K, Kankia B, Gopalakrishnan S, Yang V, Cramer E, Saladores P, Gorelick RJ, Guo J, Musier-Forsyth K, Levin JG. Fidelity of plus-strand priming requires the nucleic acid chaperone activity of HIV-1 nucleocapsid protein. Nucleic Acids Res. 2009 37:1755-1766
- Iwatani Y, Chan DSB, Liu L, Yoshii H, Shibata J, Yamamoto N, Levin JG, Gronenborn AM, Sugiura W. HIV-1 Vif-mediated ubiquitination/degradation of APOBEC3G involves four critical lysine residues in its C-terminal domain. Proc Natl Acad Sci USA. 2009 106:19539-19544.
- Wu T, Datta SAK, Mitra M, Gorelick RJ, Rein A, Levin JG. Fundamental differences between the nucleic acid chaperone activities of HIV-1 nucleocapsid protein and Gag or Gag-derived proteins: biological implications. Virology. 2010 405:556-567.
Collaborators
- Eric O. Freed, PhD, HIV Drug Resistance Program, NCI at Frederick, Frederick, MD
- Robert J. Gorelick, PhD, AIDS and Cancer Virus Program, SAIC-Frederick, Inc., NCI at Frederick, Frederick, MD
- Angela M. Gronenborn, PhD, University of Pittsburgh Medical School, Pittsburgh, PA
- Yasumasa Iwatani, PhD, National Hospital Organization Nagoya Medical Center, Nagoya, Japan
- Karin Musier-Forsyth, PhD, Ohio State University, Columbus, OH
- Alan Rein, PhD, HIV Drug Resistance Program, NCI at Frederick, Frederick, MD
- Ioulia Rouzina, PhD, University of Minnesota, Minneapolis, MN
- Klaus Strebel, PhD, Laboratory of Molecular Microbiology, NIAID, Bethesda, MD
- Shixing Tang, MD, PhD, Center for Biologics Evaluation and Research, FDA, Bethesda, MD
- Mark C. Williams, PhD, Northeastern University, Boston, MA
Contact
For more information, email levinju@mail.nih.gov or visit jlevinlab.nichd.nih.gov.