You are here: Home > Section on Developmental Biology
Molecular Genetics of Embryogenesis in Zebrafish and Xenopus
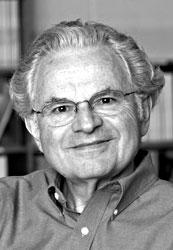
- Igor B. Dawid, PhD, Head, Section on Developmental Biology
- Mi Ha Kim, PhD, Visiting Fellow
- Minho Won, PhD, Visiting Fellow
- Valeria Zarelli, PhD, Visiting Fellow
- Alison M. Heffer, PhD, Intramural Research Training Award Fellow
- Martha Rebbert, BS, Senior Technician
- Nathaniel A. Parker, BA, Postbaccalaureate Student
- Allisan Aquilina-Beck, MS, Fish Technician
- Falicia Elenberg, Summer Intern
The laboratory uses the frog Xenopus laevis and the zebrafish Danio rerio as experimental systems in which to study molecular-genetic mechanisms of early vertebrate development. Recently, we focused on mechanisms of neural crest specification, axon guidance, cell-cell adhesion, and targeted gene disruption.
The BTB domain protein Kctd15 restricts the neural crest domain in zebrafish embryos and inhibits transcription factor AP-2.
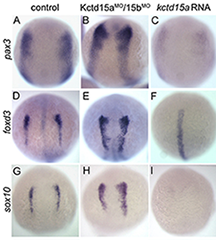
Click image to enlarge.
Figure 1. Kctd15 inhibits neural crest specification in zebrafish.
In situ hybridization of early somite embryos shows that markers of neural plate border (pax3) and neural crest (foxd3, sox10) are inhibited by Kctd15 overexpression and enhanced by its knock-down. Adapted from Dutta S, Dawid IB. Development 2010;137:3013-3018.
Our laboratory has a long-standing interest in the formation of the neural crest (NC), a group of cells with stem-cell properties that arise at the dorsal neural tube and migrate to many locations in the embryo to give rise to a large number of varied differentiated derivatives. A recent focus has been the role of the BTB domain–containing protein Kctd15 in regulating the NC domain in the embryo. Kctd15 is first expressed at the neural plate border where the NC domain is specified. Overexpression of Kctd15 strongly inhibits NC specification, whereas knock-down of Kcdt15 by antisense morpholinos enhances the expression of the neural plate border marker genes (Figure 1). We propose that Kctd15 is involved in delineating the size of the NC domain at the neural plate border.
In pursuing the molecular mechanism of Kctd15 action, we found that Kctd15 regulates the activity of transcription factor AP-2. AP-2 is known to be a key factor in the induction and differentiation of NC cells. We found that AP-2 and Kctd15 can interact when co-expressed in cultured cells. Further, Kctd15 is a highly effective inhibitor of AP-2 activity, as assayed using a reporter construct in cultured cells or in zebrafish embryos (Figure 2). In studying the mechanism of inhibition of AP-2 by Kctd15, we found that Kctd15 interacts with the activation domain of AP-2. We further analyzed this system by using a fusion product between the Gal4 DNA–binding domain and the AP-2–activation domain. Within the latter domain, a conserved proline-rich motif proved critical for Kctd15 interaction: mutation of proline 59 to alanine (P59A) in the Gal4–AP-2 fusion resulted in a protein that was active but could not bind and was insensitive to inhibition by Kctd15 (Figure 3). The proline residue was also essential for Kctd15 sensitivity in the context of the full-length AP-2 molecule. Thus, we conclude that Kctd15 inhibits AP-2 by binding to a specific site in its activation domain (1).
In additional functional studies, we determined that Kctd15 can be SUMOylated at a site close to its C terminus. SUMOylation is often associated with transcriptional repression, but we found that inhibition of AP-2 does not require SUMOylation of Kctd15. We speculate that Kctd15 carries out other biological roles that may depend on its ability to be SUMOylated (2).
Figure 2. Kcdt15 represses AP-2 function.
(A) AP2-Luc reporter, containing three AP-2 consensus sites driving expression of luciferase (Luc). (B) The reporter was strongly stimulated by zebrafish AP-2α and dramatically inhibited by zebrafish Kctd15 (levels indicated in ng). RLU, relative light units. (C) Reporter activity in zebrafish embryos. (D) Cells were transfected with FOS-tagged zebrafish AP-2α and Kctd15, and lysates were blotted to assay expression of both proteins. From Zarelli and Dawid, 2013 (1).
Figure 3. Critical role of P59 for Kctd15 interaction and inhibition of AP-2
(A) Mutants in the conserved PPxY motif within the AP-2α proline-rich domain (PRD) and two additional mutants in the adjoining region are shown. PRDs were fused to the Gal4-DNA binding domain and the FOS tag. (B) Kctd15 interaction depends on P59. WT and mutant fusion constructs were expressed in HEK293T cells alone or with Kctd15, and complexes were pulled down using a Strep-Tactin matrix. PRDs could bind to Kctd15 except in the constructs containing the P59A mutation (P59A, 4A and 6A). (C) PPxY-mutants activate the UAS-Luc reporter two-to-five fold more highly than WT PRD-Gal4. PRD-Gal4 WT and P60A, Y62A and 2A were dramatically inhibited by zebrafish Kctd15, whereas P59A and mutants containing this change were inhibited two-fold or less. The constructs were expressed at similar levels (B). Figure from Zarelli and Dawid, 2013 (1).
Habenular commissure formation in zebrafish is regulated by the pineal gland–specific gene unc119c.
We studied the pineal gland, a brain region critical for the control of circadian rhythm, by characterizating the pineal transcriptome at different stages of zebrafish development. Functional analysis of an individual gene with differential expression in the pineal gland, named unc119c, was completed, showing that the gene is required for the habenular commissure (HC) to extend across the midline. The Unc119 family is represented by two genes in the human, but the gene we isolated is the third family member noted in zebrafish. The gene, which we named unc119c, is specifically expressed in the pineal, with a low level of expression in the retina. In fish, the pineal is a photosensitive organ and shows many similarities in gene expression to the retina. Unc119 proteins interact with small GTPases of the Arl3 family, and we showed that Unc119c binds to Arl3l2 when both are coexpressed in heterologous cells. Using morpholino antisense oligonucleotide–mediated knock-down of Unc119c expression, we found that the protein is required for the formation of the HC in the zebrafish (3). The HC crosses the midline in close proximity to the pineal gland (Figure 4). Knock-down of the Unc119c–binding partners Arl3l1 or Arl3l2 also affects HC formation. We hypothesized that Unc119c may be involved in protein trafficking or secretion of a guidance factor active in HC formation. Based on the literature and expression pattern, we picked wnt4a as a candidate target gene; Wnt4a has been reported by others to be required for HC formation, a fact confirmed in our experiments. We found that Wnt4a accumulation and secretion from cultured HEK293T cells is stimulated by Unc119c and Arl3l1/2. Thus, we propose that Unc119c is required in the pineal gland to stimulate Wnt4a secretion, while Wnt4a acts in turn as a guidance cue for the HC as it traverses the zebrafish forebrain (3).
Figure 4. Unc119c is involved in habenular commissure formation in zebrafish embryos.
(A, B) Visualizing the habenular commissure (HC). Transgenic zebrafish, Tg(AANAT2:eGFP), which express eGFP in the pineal gland, were used. The embryos were fixed at 54 hours post-fertilization (hpf) and stained with anti-GFP (green, pineal gland) and anti-acetylated tubulin (red, axonal tracts). The HC is located directly beneath the pineal gland. Dorsal view (A), lateral view (B). (C-G) Injection of unc119c MO disrupts HC formation. Wild-type zebrafish embryos were injected with control MO (C), unc119c ATG MO (D), or unc119c ATG MO together with unc119c RNA (E, F). Two different unc119c splice MOs, E3I3 and I3E4, also affected HC formation in a dose-dependent manner (G). Statistical significance is indicated as * signifying p<0.05, and ** signifying p<0.01. Experiments are compared with HC in control embryos (which were all normal), except for the rescue data (F, second bar, MO+RNA), which are compared with MO-injected embryos (F, first bar). The splice MOs greatly reduced formation of mature mRNA (H). dvdt, dorsoventral diencephalic tract; hc, habenular commissure; p, pineal gland; pc, posterior commissure. From Toyama et al., 2013 (3).
Cell adhesion in zebrafish and frog embryos is modulated by March 8.
E3 ubiquitin ligases catalyze the modification of proteins by ubiquitin, a major regulatory pathway in cell physiology. Protein ubiquitination has multiple consequences, the most common and the most extensively studied being the targeting of modified proteins for degradation through the proteasome. Among the many subfamilies of E3 ubiquitin ligases, a group of trans-membrane forms of the enzyme has recently attracted much interest. March (Membrane Associated RING-CH) proteins have been studied mostly in the context of the immune system, where they have important roles, but their function in embryogenesis is largely unknown. The first family member was discovered as a cellular homolog of a viral protein involved in immunosuppression. March 8, the founding member of the family, is known to catalyze the ubiquitination and consequent downregulation of cell-surface molecules such as MHC II, B7.2 (CD86), and others. However, essentially nothing was known about possible functions of March 8 in cell types not part of the immune system, and specifically in embryonic development. We found that March 8 is expressed in the early embryo both in zebrafish and the frog Xenopus laevis, and we proceeded to study its possible functions at these stages (4). Both inhibition of expression and overexpression of March 8 proved deleterious to development. The effects of overexpression were most striking, leading to a loss of cell-cell adhesion followed by cell dissociation in zebrafish embryos and in Xenopus animal explants (so-called animal caps), where the effect could be most clearly visualized (Figure 5). In searching for a molecular basis of this cell dissociation, we noted that March 8 could bind to E-cadherin in co-immunoprecipitation experiments and that an excess of March 8 led to a reduction of cell surface E-cadherin in zebrafish embryos and in human cultured cells. Given that E-cadherin is the major cell-cell adhesion factor in the early embryo, we believe that cell dissociation by March 8 is attributable at least in part to the protein's effect on E-cadherin surface localization. We hypothesize that the biological function of March 8 in the embryo is the modulation of cell-cell adhesion, which must be maintained within a certain range to allow normal development to proceed (4).
Figure 5. March8 induces cell dissociation in Xenopus animal caps.
Xenopus embryos were injected with 400 pg of LacZ as control, Xenopus march8 or zebrafish march8 mRNA. Animal caps were dissected and incubated in a salt solution with gentle shaking. Caps were photographed at the indicated times after dissection. From Kim et al., 2014 (4).
Publications
- Zarelli VE, Dawid IB. Inhibition of neural crest formation by Kctd15 involves regulation of transcription factor AP-2. Proc Natl Acad Sci USA 2013;110:2870-2875.
- Zarelli VE, Dawid IB. The BTB-containing protein Kctd15 is SUMOylated in vivo. PLoS One 2013;8(9):e75016.
- Toyama R, Kim MH, Rebbert ML, Gonzales J, Burgess H, Dawid IB. Habenular commissure formation in zebrafish is regulated by the pineal gland-specific gene unc119c. Dev Dyn 2013;242:1033–1042.
- Kim MH, Rebbert ML, Ro H, Won M, Dawid IB. Cell adhesion in zebrafish embryos is modulated by March 8. PLoS One 2014;9(4):e94873.
- Komiya Y, Mandrekar N, Sato A, Dawid IB, Habas R. Custos controls β-catenin to regulate head development during vertebrate embryogenesis. Proc Natl Acad Sci USA 2014;111:13099-13104.
Collaborators
- Harold Burgess, PhD, Program in Genomics of Differentiation, NICHD, Bethesda, MD
- Raymond Habas, PhD, Temple University, Philadelphia, PA
- Hui Zhao, PhD, The Chinese University, Hong Kong, China
Contact
For more information, email dawidi@mail.nih.gov or visit sdb.nichd.nih.gov.