You are here: Home > Section on Molecular Regulation
Global Regulation of Gene Expression by ppGpp
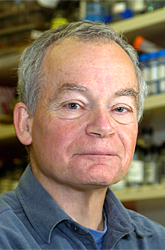
- Michael Cashel, MD, PhD, Head, Section on Molecular Regulation
- Katarzyna Potrykus, PhD, Research Fellow
Two naturally occurring nucleotide regulators, abbreviated as (p)ppGpp, have been studied in our lab for many years. These are analogs of GTP and GDP with 3′ pyrophosphate residues. They function as important second messengers in bacteria, plants, and now arguably in animal cells, including humans. It is clear that many sources of nutritional and environmental stress result in diverse metabolic imbalances that signal increased (p)ppGpp, which in turn provokes massive adjustments of global gene expression at the transcriptional level. However provoked, the adjustments are essential for the biological survival of all organisms (1). Invasive bacteria that survive the many challenges elaborated by host defenses are pathogens, by definition. It thus comes as no surprise that (p)ppGpp regulators play key roles to enhance the virulence of nearly all pathogens, which provide fundamentally new avenues for antibiotic development, with potentially important implications for the control of resistant pathogens. It is relevant that, until recently, (p)ppGpp was thought absent in animals. Another research group discovered that enzymes functioning in (p)ppGpp metabolism are present throughout the animal kingdom. The enzymes are hydrolases that can cleave (p)ppGpp and are encoded by genes called Mesh. The Mesh hydrolases are such close structural and catalytic homologues of bacterial hydrolases that they function interchangeably in animal and bacterial cells. A role for these genes in flies is suggested because their deletion perturbs embryogenesis during nutrient limitation, leading to altered transcriptional profiles reminiscent of bacterial regulation. Nevertheless (p)ppGpp has not been documented in animals—not in itself definitive evidence of its absence, given that identification of (p)ppGpp is extraordinarily difficult. The new goal of our section is to search for (p)ppGpp or (p)ppGpp–like regulatory systems using approaches that exploit our microbiological experience with (p)ppGpp. This work is carried out in parallel with our longstanding efforts to understand the details of global effects of ppGpp on gene expression and transcription using microbial genetics in E. coli as the most tractable approach (2, 3, 4, 5).
The search for new (p)ppGpp-like regulators
Direct identification of the ppGpp nucleotide in animal cells is difficult despite the high sensitivity of currently available chemical techniques; for example, it has eluded quantitation by mass-spectrometric metabolomic approaches even in bacteria where ppGpp can reach levels as high as those of GTP, in part owing to the extreme metabolic lability of ppGpp (a half life in bacteria of about 20 sec) coupled with its chemical instability. So far, (p)ppGpp has not been found in eukaryotes. If (p)ppGpp is indeed absent, it is possible the Mesh1 hydrolases function to degrade some compound other than (p)ppGpp that shares structural similarity to (p)ppGpp. We have embarked on structure-specificity studies with purified Mesh proteins in search of possible candidates that could provide clues to functions neutralized by Mesh.
Our hypothesis is that regulatory ppGpp-like products exist in various organisms, which have a hydrolase with a substrate specificity matching that for (p)ppGpp. In support of this idea, we recently found that classical animal Mesh hydrolases do display more extended substrate specificities than do bacteria. Bacterial hydrolases attack the ribose 3′-pyrophosphoryl groups of 6-hydroxy purines [(p)ppGpp and (p)ppIpp] but not pppApp or ppApp. In contrast, the human and fly Mesh hydrolases are also able to cleave otherwise similar adenine analogs. As mentioned above, we elected to look for the corresponding synthetases in more experimentally accessible bacterial systems than in animal cells. The genomes of a small fraction (0.07) of nearly 1,000 species encode a few very close homologs of animal Mesh hydrolases, in addition to their standard (p)ppGpp–specific hydrolase. Our approach is to first find out whether these unusual bacterial hydrolase homologues share the degenerate substrate specificity of the animal enzymes. If they do, the structural determinants that extended catalytic specificity will be explored and the corresponding synthetases will be cloned, purified, and studied to determine whether synthetic products indeed match those of the hydrolase. The regulatory effects any new ppGpp-like regulators will be studied in modified E. coli cells that have been constructed to allow systematic manipulation of the abundance of regulator nucleotides, as we have done for ppGpp and pppGpp.
Regulatory effects of ppGpp on transcription
We seek to understand how ppGpp regulates gene expression through promoter-specific positive and negative regulatory effects on transcription. For years we have focused on regulatory interactions between RNAP secondary channel–binding proteins (such as DksA, GreA, GreB) and ppGpp. Our recent analyses (5) confirm expectations from structural similarities and parallel channel binding to RNA polymerase that DksA and GreA/B can show similar and even redundant functions. One example is that GreA overproduction can override the amino acid requirements of a dksA mutant for growth in minimal medium (ILTV). These requirements are a subset of ppGpp0 requirements (DEFHILSTV). A related example is that GreA or DksA overexpression can reverse some of the amino acid requirements shown by ppGpp0 cells. We used microarray transcription profiles to extend earlier findings of shared functions by GreA and DksA to the cellular transcriptome. This more detailed global analysis reveals instances of GreA and DksA acting in opposition. An example of opposite effects at the phenotypic level is that the complete reversal of the amino amino acid requirements of ppGpp0 cells by overexpression of GreA occurs only in the absence of DksA and vice versa. This surprising observation suggests that the presence of one protein is redundant to the effect of the other gene product but abolished by the simultaneous presence of both. This oddly symmetrical epistatic effect is reminiscent of synthetic gene lethals.
While redundancies exist, the effects of these gene products are not quantitatively equivalent. As judged by transcription profiling, more genes (331) are affected by GreA overproduction when DksA is absent than when DksA is present (45), yet genes affected by DksA when GreA is absent show a very modest skew. Profiling indicates that the strongest activation by GreA occurs for gadA and gadE genes. We could verify this with PgadA– and PgadE–lacZ promoter transcriptional fusions as reporters, which serve to again localize activation to the level of transcription initiation rather than elongation. To determine what functional attributes of GreA and DksA are responsible for their interactive effects on regulation, we used well known mutants of GreA and DksA with altered acidic residues in the tip of the coil-coil finger structure. When GreA or DksA is bound to RNA polymerase, the fingertip reaches through a secondary channel deep into RNA polymerase to reach a point near its catalytic center for transcription. There, the fingertip acidic residues are thought to provoke the classical effects of such factors on RNA chain elongation and relief of arrested elongation. While the mutants do abolish the classical functions, they do not alter the regulation of selected promoter-lacZ fusions. This surprising observation strongly suggests that the regulatory features we uncovered represent new phenomena not easily explained by known behavior.
These more detailed approaches reinforce our notions from earlier experiments that the effects of ppGpp involve a complex interplay between GreA, GreB, and DksA. Stated in another way, it can be argued that our experiments reveal a modulatory function for ppGpp for this interplay, which could be the primary cause of complex global changes in gene expression. Mechanistically, this could occur by promoter activation, competition for RNA polymerase at the level of either binding, secondary channel occupancy, as well as mutual control of the expression of other secondary channel proteins. Our transcriptional profiling studies serve to localize some of the interactions to specific genes and regulators, which might lead to new regulatory mechanisms at the molecular level. We also explored the interactions between the three factors in a hierarchical series of RNAP mutants of increasing severity that allow ppGpp0 strains to grow without amino acids. The work again confirms that the ppGpp dependence of factor interactions operates at the level of RNAP—unusual for bacteria, for which gene-specific regulation otherwise almost invariably involves proteins that recognize specific DNA sequences. Overall, our experiments provide evidence that ppGpp regulation operates through a more complex regulatory interplay between GreA, GreB, and DksA than has been appreciated.
Publications
- Szalewska-Palasz A, Potrykus K, Cashel M, Wegrzyn G. Starvation of bacteria for amino acids as an example of prokaryotic response to nutritional deprivation. In: Merkin TC, ed. Biology of Starvation in Humans and Other Organisms, Nova Science Publishers, New York 2011;Chapter 4.
- James T, Cashel M, Hinton D. A mutation within the beta subunit of Escherichia coli RNA polymerase impairs transcription from bacteriophage T4 middle promoters. J Bacteriol 2011;192:5580-5587.
- Potrykus K, Murphy M, Philippe N, Cashel M. ppGpp is the major source of growth rate control in E. coli. Environ Microbiol 2011;13:563-576.
- Edwards AN, Paterson-Fortin LM, Vakulskas CA, Mercante JW, Potrykus K, Vinella D, Comancho MI, Fields JA, Thompson SA, Georgellis D, Cashel M, Babitzke P, Romeo T. Circuitry linking the Csr and stringent response global regulatory systems. Mol Microbiol 2011;80:1561-1580.
- Vinella D, Potrykus K, Murphy H, Cashel M. Effects on growth by changes of the balance between GreA, GreB and DksA suggest mutual compeitition and functional redundancy in Escherichia coli. J Bacteriol 2012;194:261-273.
Contact
For more information, email cashel@mail.nih.gov or visit smr.nichd.nih.gov.