You are here: Home > Section on Neural Developmental Dynamics
Building the Zebrafish Lateral Line System
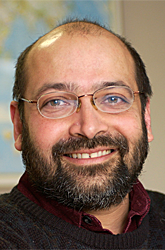
- Ajay Chitnis, MBBS, PhD, Head, Section on Neural Developmental Dynamics
- Damian E. Dalle Nogare, PhD, Postdoctoral Fellow
- Miho Matsuda, PhD, Postdoctoral Fellow
- Kyeong-Won Yoo, PhD, Postdoctoral Fellow
- Gregory Palardy, BS, Research Technician
- Chongmin Wang, MS, Research Technician
- Jeffery Head, BS, Postbaccalaureate Intramural Research Training Award Fellow
- Katherine Somers, BS, Postbaccalaureate Intramural Research Training Award Fellow
- Tara Srirangarajan, Summer Student
Our goal is to understand how the architecture of the mature nervous system emerges as a consequence of local interactions between cells during early development. We use a combination of cellular, molecular, genetic, and computational tools to understand how cells differentiate in distinct patterns in the various compartments of the zebrafish nervous system. We analyze zebrafish mutants and embryos microinjected with morpholinos or mRNA to alter gene function. We examine mechanisms involved in the division of the prospective neural tissue into compartments with distinct fates and examine how cell differentiation is regulated within each. Our current studies examine how the posterior lateral line system is built in the zebrafish nervous system. Our goal is to define the gene-regulatory network that coordinates cell fate and morphogenesis in the lateral line system and to build computational models, based on these studies, that help us understand how this relatively simple and extremely accessible sensory system in zebrafish builds itself.
The lateral line is a mechanosensory system that detects water flow and consists of sensory organs called neuromasts, which are distributed in a stereotypical pattern over the surface of the zebrafish. Each neuromast has sensory hair cells at its center. The hair cells are surrounded by support cells, which serve as progenitors for the production of more hair cells during growth and regeneration of neuromasts. The development of this superficial sensory system in zebrafish can be observed easily in live embryos with transgenic lines expressing fluorescent proteins in specific subsets of cells of the lateral line system. In addition, a range of genetic and cellular manipulations can be used to investigate gene function.
The function of sensory hair cells in fish neuromasts is remarkably similar to that of hair cells in the vertebrate ear. In addition, the gene-regulatory network that determines specification of neuromast hair cells is very similar to the one specifying hair cell fate in our ear. Like the hair cells in our ears, neuromast hair cells can be damaged by exposure to drugs such as aminoglycosides and to copper ions and noise. However, unlike our ears, in which loss of hair cells can be permanent, neuromast hair cells have the remarkable ability to regenerate. Hence, the lateral line system serves as an excellent model system with which to understand development and for developing strategies for engineering regeneration of sensory hair cells.
The posterior lateral line system is initially established by the posterior lateral line primordium (PLLp), a cluster of about a 100 cells that migrates from the ear to the tip of the tail periodically depositing neuromasts. Recent studies showed that the mechanisms determining and guiding collective migration and deposition of cells from the pllp are remarkably similar to those determining the collective migration of metastatic cancer cells. Hence, the lateral line system has also recently emerged as an excellent system for studying the biology of metastatic cancer cells.
Our expectation is that understanding the gene-regulatory network that coordinates cell fate and morphogenesis of the zebrafish lateral line system will ultimately have a profound impact on translational studies that address a wide range of issues including the development and regeneration of sensory systems and therapies directed at limiting the spread of cancer through metastasis.
Lef1 regulates neuromast formation and spacing by determining expression of the FGF inhibitor Dusp6 in the leading domain of the posterior lateral line primordium.
The posterior lateral line primordium (PLLp) migrates caudally, periodically depositing neuromasts. The migrating PLLp contains 2–3 immature neuromasts or proto-neuromasts at various stages of maturation. Their formation is periodically initiated toward the leading end of the PLLp. As they mature, they are deposited from the trailing end of the migrating PLLp. While previous studies showed that FGF signaling initiates proto-neuromast formation in the PLLp, mechanisms that determine their periodic formation toward the leading end and deposition from the trailing end remain poorly understood.
FGF receptor signaling is initiated by FGFs expressed in response to Wnt signaling in the PLLp. Initially, there is Wnt activity along the entire length of the PLLp; however, it is strongest toward the leading end of the PLLp. Wnt signaling locally inhibits FGF receptor activation, so that FGFs expressed in response to Wnt signaling in a leading domain first initiate FGF signaling and proto-neuromast formation at the trailing end of the PLLp. Given that FGF signaling, associated with formation of proto-neuromasts, also inhibits Wnt signaling, periodic proto-neuromast formation is accompanied by restriction of Wnt activity, defined by a domain of lef1 expression, to a progressively smaller leading domain of the PLLp. We now have evidence that the progressive shrinking of the lef1 domain may coordinate systematic changes in the behavior and fate of cells in the migrating PLLp, leading to PLLp's eventual loss and to the termination of the PLLp system.
Cells in the leading part of the migrating PLLp move in a cohesive column as they undergo collective migration. However, as they enter a specific part of the trailing domain they slow down in preparation for deposition. Previous studies by Aman et al. suggested that homeostatic mechanisms keep the length of the leading cohesive column relatively constant. In the context of such a regulatory mechanism, it was suggested that proliferation within the PLLp determines when cells are displaced into a trailing domain, where they lose their migratory behavior in preparation for deposition. Based on this hypothesis, it was suggested that reduction in cell division or manipulations that increase cell death slow the displacement of cells into the trailing domain, providing a possible explanation for the delay in deposition and the increase in neuromast spacing following manipulations that reduce cell division or increase cell death.
It was also shown that both Wnt and FGF signaling determine proliferation in the PLLp. Lef1 is a mediator of Wnt signaling, and its loss reduces proliferation in the PLLp. However, in lef1–deficient embryos, neuromasts are deposited closer together, and the PLLp terminates prematurely. Valdivia et al. suggested that, contrary to the expectations of the model described above, this results from reduced proliferation limited to the leading domain of the PLLp, specifically accelerating the shrinking of the Wnt system. Furthermore, they suggested that the Wnt system secretes a factor that determines the length of the cohesive column of cells undergoing collective migration. In this context, they suggested, accelerated shrinking of the Wnt system leads to accelerated deposition, accounting for closer spacing and premature termination of the PLLp system. McGraw et al. suggested an alternate explanation for the premature termination of the PLLp system in lef1 mutants, namely, that it was due to premature incorporation of progenitors into proto-neuromasts. However, they did not provide an explanation for the altered spacing of deposited neuromasts.
We found that rspo3 knockdown reduces proliferation in a manner similar to that seen in lef1 morphants but does not cause closer neuromast deposition or premature termination of the PLLp, suggesting that the changes in lef1–deficient embryos are not linked to changes in proliferation. Instead, we suggest that they are related to Lef1's role in regulating the balance of Wnt and FGF functions in the PLLp. By determining expression of an FGF signaling inhibitor, Dusp6, in leading cells, Lef1 regulates incorporation of cells into neuromasts; reduction of Dusp6 expression in leading cells in lef1–deficient embryos allows new proto-neuromasts to form closer to the leading edge, slowing PLLp migration, reducing spacing between deposited neuromasts, and allowing premature termination of the PLLp system.
While the lateral line system serves as a unique model for studying the self-organization and the potential for the regeneration of a sensory system, it also serves as a highly effective system in which to investigate mechanisms regulating collective migration of cells and regulating growth and differentiation. Study of the lateral line system has already shown that, during normal development of this relatively simple system, such mechanisms bear a striking resemblance to those that determine unregulated growth and metastasis in cancer. Hence our studies are expected to provide insight into mechanisms that play a key role in both development and disease.
Building working models of the migrating posterior lateral line primordium—models and experiment
Previous studies provided a remarkable framework for understanding how chemokine signaling steers the PLLp and how juxtaposed mutually inhibitory Wnt and FGF signaling systems coordinate cell fate and morphogenesis of proto-neuromasts in the PLLp. We built computational models of the PLLp based on this framework using Netlogo, an agent-based modeling platform, to recapitulate the self-organization of this system. The models show that, while the current framework helps to account for many of the emergent properties of the PLLp, several questions remain unanswered. One of the models is a "mechanical" model of the PLLp based on current understanding of how chemokine signals guide PLLp migration. The model can account for the complex behavior of leading cells when a fragment of the PLLp is severed from trailing cells of the PLLp. However, its failure to recapitulate some behaviors of trailing cells helped define gaps in our understanding of how trailing cells follow leading cells, which led to new experiments and a surprisingly simple new model that shows how trailing cells follow leading cells during collective migration of cells in the PLLp.
Additional Funding
- K99 award to Miho Matsuda
Publications
- Chitnis AB, Nogare DD, Matsuda M. Building the posterior lateral line system in zebrafish. Dev Neurobiol 2012;72(3):234-255.
- Ball ER, Matsuda MM, Dye L, Hoffmann V, Zerfas PM, Szarek E, Rich A, Chitnis AB, Stratakis CA. Ultra-structural identification of interstitial cells of Cajal in the zebrafish Danio rerio. Cell Tissue Res 2012;349(2):483-491.
- York AG, Parekh SH, Dalle Nogare D, Fischer RS, Temprine K, Mione M, Chitnis AB, Combs CA, Shroff H. Resolution doubling in live, multicellular organisms via multifocal structured illumination microscopy. Nat Methods 2012;9(7):749-754.
- Dong Z, Yang N, Yeo SY, Chitnis A, Guo S. Intralineage directional Notch signaling regulates self-renewal and differentiation of asymmetrically dividing radial glia. Neuron 2012;74(1):65-78.
- Veleri S, Bishop K, Dalle Nogare DE, English MA, Foskett TJ, Chitnis A, Sood R, Liu P, Swaroop A. Knockdown of Bardet-Biedl syndrome gene BBS9/PTHB1 leads to cilia defects. PLoS One 2012;7(3):e34389.
Collaborators
- Su Guo, PhD, University of California San Francisco, San Francisco, CA
- Hari Shroff, PhD, Laboratory of Molecular Imaging and Nanomedicine, NIBIB, Bethesda, MD
- Constantine Stratakis, MD, DSc, Program in Developmental Endocrinology and Genetics, NICHD, Bethesda, MD
- Anand Swaroop, PhD, Neurobiology Neurodegeneration & Repair Laboratory, NEI, Bethesda, MD
Contact
For more information, email chitnisa@mail.nih.gov.