You are here: Home > Unit on Behavioral Neurogenetics
Neuronal Circuits Controlling Behavior: Genetic Analysis in Zebrafish

- Harold Burgess, PhD, Head, Unit on Behavioral Neurogenetics
- Sadie A. Bergeron, PhD, Postdoctoral Fellow
- Kandice Fero, PhD, Postdoctoral Fellow
- Tohei Yokogawa, PhD, Postdoctoral Fellow
- Hiba Codore, BSc, Zebrafish Technician
- Markus C. Hannan, Postbaccalaureate Fellow
- Daniel Valenzuela, Summer Student
Our goal is to understand how neuronal circuits in larval zebrafish produce appropriate motor responses under diverse environmental contexts. Locomotor behavior in zebrafish larvae is controlled by neuronal circuits that are established through genetic interactions during development. We aim to identify genes and neurons that are required for the construction and function of the brainstem circuits underlying specific behaviors. Neuronal circuits situated in the brainstem form the core of the locomotor control network in vertebrates and are responsible for balance, posture, motor control, and arousal. Accordingly, many neurological disorders stem from abnormal formation or function of brainstem circuits. Insights into the function of brainstem circuits in health and disease have come from genetic manipulation of neurons in zebrafish larvae, in combination with computational analysis of behavior.
Several unique features of the zebrafish model system facilitate analysis of the neuronal basis of vertebrate behavior. The larval zebrafish brain exhibits the basic architecture of the vertebrate brain but is much less complex than the mammalian brain. The optical clarity of the embryo facilitates visualization of individual neurons and their manipulation with genetic techniques. Behavior in larvae is innate and therefore exhibits minimal variability between fish. Subtle alterations in behavior can therefore be robustly scored, making it possible to assess quickly the contribution of identified neurons to a variety of motor behaviors. We use two major behavioral paradigms to investigate the neuronal basis of vertebrate behavior: modulation of the acoustic startle response by prepulse inhibition and modulation of the locomotor repertoire during a phototaxis-based navigational task. In addition, we are developing a suite of genetic tools and behavioral assays to probe the nexus between neuronal function and behavior at single-cell resolution.
Functional mapping of serotonergic neuronal architecture
Many neurological conditions include impairments in arousal, but the complexity of the neuronal systems subserving arousal in mammals has made it difficult to understand precisely how various neuromodulator systems contribute to its control. We set out to establish a robust system for inducing arousal in zebrafish larvae. One hallmark of arousal states is elevated motor activity that persists beyond the stimulus inducing the arousal state. We speculated the the visual motor response—hyperactivity triggered by sudden darkness—might represent an arousal state in zebrafish. While performing a detailed behavioral characterization of this response, we found that the visual behavior did not require classical light-sensing organs, the eyes and pineal. Further investigation demonstrated that the behavior is triggered by deep brain photoreceptors, melanopsin-expressing neurons in the preoptic hypothalamus (1).
In a second approach to investigating arousal, we studied the function of the serotonergic system. The serotonergic system is a major neurotransmitter system modulating arousal in higher vertebrates. To ascertain the role of this system in behavioral control in larval zebrafish, we generated transgenic fish expressing the Gal4 transcription factor in serotonergic neurons. We can thus genetically manipulate the neurons by crossing the fish to lines carrying reporter genes under the control of the UAS promoter. We found that exposure to water flow induces a short-term state of arousal characterized by increased locomotor activity and sensitivity to an optomotor stimulus. Ablation of the serotonergic neurons of the dorsal raphe impairs sensory responsiveness during flow-induced arousal. We performed in vivo calcium imaging of serotonergic neurons, by injecting the Gal4 transgenic fish with a UAS:GCaMP element, and found that subsets of dorsal raphe neurons are active during flow-induced arousal. High-resolution neuronal tracing using genetic mosaic techniques demonstrated that neurons of the dorsal raphe project to the optic tectum, a likely site for the modulation of sensory responsiveness to visual cues (2). We are continuing to use a combination of approaches to correlate the connections of single serotonergic neurons with their behavioral role, establishing a neuronal-level functional map of serotonergic anatomy.
Modulation of the acoustic startle response
Previously, we demonstrated that intense acoustic stimuli elicit two types of startle response in zebrafish larvae: rapid short-latency responses and lower-performance long-latency responses. All fish can generate both types of response, but the responses remain unpredictable from trial to trial. Our goal is to understand the mechanisms that determine whether a larva deploys a short- or long-latency response. In earlier work, we found that the short-latency responses are modulated in a similar fashion to startle responses in mammals, in which startle magnitude is inhibited when the startle stimulus is preceded by a weak auditory prepulse. This form of startle modulation, termed prepulse inhibition, is diminished in several neurological conditions, including schizophrenia. Previously, we conducted a screen to identify fish carrying genetic mutations resulting in a reduction in prepulse inhibition. Using these fish, we are performing linkage analysis to map the genetic mutations in the mutants and identify genes required for prepulse inhibition. The experiments will reveal circuit components that selectively suppress generation of short-latency responses. In parallel, we are analyzing how long-latency responses are modulated. Brainstem neurons that trigger a motor response must belong to the restricted cohort of neurons that project to the spinal cord. We are therefore sequentially ablating neurons of this class by using a pulsed nitrogen laser and then probing the stimulus threshold and magnitude of the long-latency startle response system. In parallel we are performing a circuit-breaking screen, ablating a collection of brain-specific enhancer trap lines to identify neurons that modulate startle responses. Together, these approaches will allow us to find neuronal mechanisms for the implementation of behavioral choice during startle responses in zebrafish larvae.
Figure 1. Enhancer trap lines for neurobehavioral research
Examples of transgenic Gal4 enhancer trap lines. Gal4 expression is visualized by crossing lines to a fluorescent UAS:Kaede reporter. All images are dorsal views of the brain, taking advantage of the transparency of larva. Lines with broad expression in the nervous system are recovered along with lines labeling specific structures and lines with very restricted patterns of expression.
Tools for analyzing neuronal circuits that control behavior
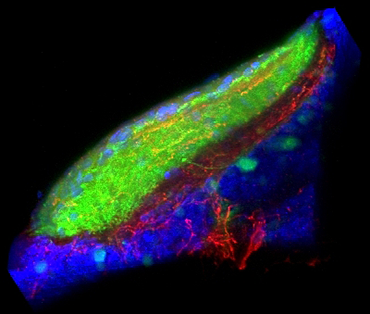
Figure 2. Sensory-motivational integration in the optic tectum
Our results implicate the otic tectum (the zebrafish homolog of the superior colliculus) as a site where motivational information modulates sensory input. Retinal ganglion fibres (green) convey visual information to the tectum, where they overlap with fibres from dorsal raphe neurons, which signal arousal (red). Counterstain for cell nuclei (blue).
The relatively simple nervous system of zebrafish larvae and the larva's restricted range of motor behaviors make it possible to identify neuronal pathways that underlie the entire behavioral repertoire. However, it would be extremely useful to have reporter lines that would permit the manipulation of small groups of neurons known to be involved in a particular motor behavior. Many existing transgenic lines labeling neurons exist, but most show expression outside the brain, limiting the usefulness of these lines for behavioral analysis in unrestrained fish. We developed a technique to restrict reporter gene expression to the CNS by adapting the neuronal-restrictive silencing element (NRSE/RE1) then performed an enhancer trap screen, creating a library of 240 Gal4 enhancer-trap transgenic fish with unique and restricted patterns of neuronal expression (Figure 1). The lines constitute a unique resource for decoding the developmental genetics and neuro-anatomical basis of behavior in zebrafish larvae. We are currently using the lines to ablate the trapped neuronal cohort and screen for defects in a broad range of behaviors, including prepulse inhibition of the startle response. Our work represents the first application of such a screen in a vertebrate organism.
Additional Funding
- Director's Challenge Innovation Award (2011) "Volumetric Imaging of Neuronal Dynamics in the Developing Zebrafish Larva" with Dr. Shroff (NIBIB)
- Japan Society for the Promotion of Science Fellowship to Dr. Yokogawa
Publications
- Fernandes AM, Fero K, Arrenberg AB, Bergeron SA, Driever W, Burgess HA. Deep brain photoreceptors control light seeking behavior in zebrafish larvae. Curr Biol 2012;22:1-6.
- Yokogawa T, Hannan MC, Burgess HA. The dorsal raphe modulates sensory responsiveness during arousal in zebrafish. J Neurosci 2012;in press.
- EauClaire SF, Cui S, Ma L, Matous J, Marlow FL, Gupta T, Burgess HA, Abrams EW, Kapp LD, Granato M, Mullins MC, Matthews RP. Mutations in vacuolar H+-ATPase subunits lead to biliary developmental defects in zebrafish. Dev Biol 2012;365:434-444.
- Jain RA, Wolman MA, Schmidt L, Burgess HA, Granato M. Molecular-genetic mapping of zebrafish mutants with variable phenotypic penetrance. PLoS One 2012;6:e26510.
- Fero K, Yokogawa T, Burgess HA. The behavioral repertoire of larval zebrafish. In: Kalueff AV, Cachat JM, eds. Zebrafish Models in Neurobehavioral Research. Springer, 2011;249-291.
Collaborators
- Wolfgang Driever, PhD, Universität Freiburg, Freiburg, Germany
- Fumihito Ono, PhD, Laboratory of Molecular Physiology, NIAAA, Rockville, MD
- Hari Shroff, PhD, Section on High Resolution Optical Imaging, NIBIB, Bethesda, MD
- David Valle, MD, The Johns Hopkins University School of Medicine, Baltimore, MD
Contact
For more information, email haroldburgess@mail.nih.gov or visit ubn.nichd.nih.gov.