You are here: Home > Section on Neural Developmental Dynamics
Building the Zebrafish Lateral Line System
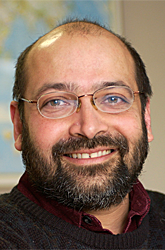
- Ajay Chitnis, MBBS, PhD, Head, Section on Neural Developmental Dynamics
- Damian E. Dalle Nogare, PhD, Postdoctoral Fellow
- Miho Matsuda, PhD, Postdoctoral Fellow
- Kyeong-Won Yoo, PhD, Postdoctoral Fellow
- Gregory Palardy, BS, Research Technician
- Chongmin Wang, MS, Research Technician
- Katherine Somers, BS, Postbaccalaureate Intramural Research Training Award Fellow
- Tara Srirangarajan, Summer Student
Our goal is to understand how the architecture of the mature nervous system emerges as a consequence of local interactions between cells during early development. We use a combination of cellular, molecular, genetic, and computational tools to understand how cells differentiate in distinct patterns in the various compartments of the zebrafish nervous system. We analyze zebrafish mutants and embryos microinjected with morpholinos or mRNA to alter gene function. We examine mechanisms involved in the division of the prospective neural tissue into compartments with distinct fates and examine how cell differentiation is regulated within each. Our current studies examine how the posterior lateral line system is built in the zebrafish nervous system. Our goal is to define the genetic regulatory network that coordinates cell fate and morphogenesis in the lateral line system and to build computational models, based on these studies, that help us understand how this relatively simple and extremely accessible sensory system in zebrafish builds itself.
The lateral line is a mechanosensory system that detects water flow and consists of sensory organs called neuromasts, which are distributed in a stereotypic pattern over the surface of the zebrafish. Each neuromast has sensory hair cells at its center. The hair cells are surrounded by support cells, which serve as progenitors for the production of more hair cells during growth and regeneration of neuromasts. The development of this superficial sensory system in zebrafish can be observed easily in live embryos with transgenic lines expressing fluorescent proteins in specific subsets of cells of the lateral line system. In addition, a range of genetic and cellular manipulations can be used to investigate gene function.
The function of sensory hair cells in fish neuromasts is remarkably similar to that of hair cells in the vertebrate ear. In addition, the gene-regulatory network that determines specification of neuromast hair cells is very similar to the one specifying hair cell fate in our ear. Like the hair cells in our ears, neuromast hair cells can be damaged by exposure to drugs like aminoglycosides, copper ions, and by exposure to noise. However, unlike our ears, in which loss of hair cells can be permanent, neuromast hair cells have the remarkable ability to regenerate. Hence, the lateral line system serves as an excellent model system for understanding development and developing strategies for engineering regeneration of sensory hair cells.
The posterior lateral line system is initially established by the posterior lateral line primordium (pllp), a cluster of about a 100 cells that migrates from the ear to the tip of the tail periodically depositing neuromasts. Recent studies have shown that the mechanisms that determine and guide collective migration and deposition of cells from the pllp are remarkably similar to those that determine the collective migration of metastatic cancer cells. Hence, the lateral line system has also recently emerged as an excellent system for studying the biology of metastatic cancer cells.
Our expectation is that understanding the genetic regulatory network that coordinates cell fate and morphogenesis of the zebrafish lateral line system will ultimately have a profound impact on translational studies that address a wide range of issues including the development and regeneration of sensory systems and therapies directed at limiting the spread of cancer through metastasis.
The balance of Wnt and FGF signaling influences the rate of neuromast deposition.
The migrating pLLP contains two to three "proto-neuromasts" at a progressive stage of maturation. Previous studies have suggested that their formation is induced near the leading end of the pLLP by FGF signaling, which in turn is dependent on the FGF ligands FGF3 and FGF10; FGF3 and FGF10 are secreted in response to Wnt signaling at the leading end of the pLLP. While Wnt signaling drives expression of fgf10 and fgf10 at the leading end of the pLLP, it simultaneously inhibits local FGF signaling by driving expression of sef, which encodes an intracellular inhibitor of FGF signaling. As a consequence, FGF ligands expressed in a leading domain can only effectively activate FGF signaling and initiate proto-neuromast formation in an adjacent trailing domain, where there is less inhibition by Wnt-dependent Sef expression. Cell proliferation adds cells to the leading end of the pLLP, progressively displacing the newly formed proto-neuromast to a more trailing position, where the proto-neuromast continues to mature. Once the leading end has elongated sufficiently, formation of another proto-neuromast is initiated. In this manner, the migrating pLLP acquires two to three proto-neuromasts at progressive stage of maturation and eventually the most mature proto-neuromast is deposited from the trailing end.
Previous studies emphasized that proliferation is a key determinant of the rate of neuromast deposition, as it determines how quickly maturing proto-neuromasts reach a trailing compartment of the pLLP, where expression of the chemokine receptor cxcr7b correlates with the slowing and eventual deposition of cells in the pLLP. However, we had observed that, as the pLLP moves from the ear to toward the tip of the tail, the Wnt-active domain progressively becomes smaller and that this correlates with neuromasts being deposited more frequently, raising the possibility that progressive changes in the balance of Wnt and FGF signaling within the migrating pLLP might also influence the rate of neuromast deposition.
To test this hypothesis we used various manipulations to alter the balance of Wnt and FGF signaling in the migrating pLLP and examined how they influence the pattern of neuromast deposition. We used a small molecule inhibitor of Wnt signaling, IWR, to reduce Wnt signaling and knocked down fgf10 function to reduce FGF signaling system, which we expected would indirectly allow expansion of the Wnt signaling system. We found that inhibition of the Wnt system with IWR accelerated proto-neuromast maturation and increased the rate of neuromast deposition. On the other hand, knockdown of fgf10 significantly delayed the establishment of a robust trailing FGF signaling system and allowed persistent and expanded Wnt signaling along the entire length of the pLLP for a significant period after its migration had started, thus delaying initiation of proto-neuromast formation and neuromast deposition. However, once the FGF system was established by the remaining FGF ligand, FGF3, the Wnt active domain began to shrink, proto-neuromast formation was initiated, and the pLLP began depositing neuromasts. Interestingly, once the Wnt system began to shrink in fgf10–morphant embryos, it shrank faster than in control embryos in which the balance of Wnt and FGF system had not been disturbed. This relatively rapid shrinking of the Wnt active domain correlated with a faster rate of neuromast deposition. Together, these observations show that balance of Wnt and FGF signaling is critical in determining when formation of new proto-neuromasts is initiated, how quickly they mature, and when they are eventually deposited.
Previous studies had indicated that the rate of neuromast deposition is determined by the rate of cell proliferation and that Wnt signaling is an important determinant of cell proliferation. However, other studies examining the role of Lef1 in determining Wnt-dependent cell proliferation suggested that loss of lef1 function results in an accelerated rate of neuromast deposition, just as we had seen following inhibition of Wnt signaling with IWR. To resolve the paradox of why loss of Lef1, which clearly reduced proliferation, was not reducing the rate of neuromast deposition, we hypothesized that loss of Lef1 may both reduce proliferation and have other independent effects that accelerate neuromast formation and deposition.
Additional Funding
- K99 award to Miho Matsuda
Publications
- Chitnis AB, Nogare DD, Matsuda M. Building the posterior lateral line system in zebrafish. Dev Neurobiol 2011;[Epub ahead of print].
Contact
For more information, email chitnisa@mail.nih.gov.