You are here: Home > Unit on Behavioral Neurogenetics
Neuronal Circuits Controlling Behavior: Genetic Analysis in Zebrafish
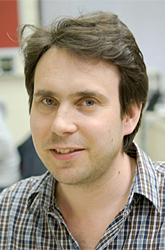
- Harold Burgess, PhD, Head, Unit on Behavioral Neurogenetics
- Sadie A. Bergeron, PhD, Postdoctoral Fellow
- Kandice Fero, PhD, Postdoctoral Fellow
- Tohei Yokogawa, PhD, Postdoctoral Fellow
- Hiba Codore, BSc, Zebrafish Technician
- Markus C. Hannan, Postbaccalaureate Fellow
- Alex Wendling, Summer Student
Our goal is to understand how neuronal circuits in larval zebrafish produce appropriate motor responses under diverse environmental contexts. Locomotor behavior in zebrafish larvae is controlled by neuronal circuits that are established through genetic interactions during development. We aim to identify genes and neurons that are required for the construction and function of the brainstem circuits underlying specific behaviors. Neuronal circuits situated in the brainstem form the core of the locomotor control network in vertebrates and are responsible for balance, posture, motor control, and arousal. Accordingly, many neurological disorders stem from abnormal formation or function of brainstem circuits. Insights into the function of brainstem circuits in health and disease have come from genetic manipulation of neurons in zebrafish larvae, in combination with computational analysis of behavior.
Several unique features of the zebrafish model system facilitate analysis of the neuronal basis of vertebrate behavior. The larval zebrafish brain exhibits the basic architecture of the vertebrate brain but is much less complex than the mammalian brain. The optical clarity of the embryo facilitates visualization of individual neurons and their manipulation with genetic techniques. Behavior in larvae is innate and therefore exhibits minimal variability between fish. Subtle alterations in behavior can therefore be robustly scored, making it possible to assess quickly the contribution of identified neurons to a variety of motor behaviors. We use two major behavioral paradigms to investigate the neuronal basis of vertebrate behavior: modulation of the acoustic startle response by prepulse inhibition and modulation of the locomotor repertoire during a phototaxis-based navigational task. In addition, we are developing a suite of genetic tools and behavioral assays to probe the nexus between neuronal function and behavior at single-cell resolution.
Modulation of the acoustic startle response
Previously, we demonstrated that intense acoustic stimuli elicit two types of startle response in zebrafish larvae: rapid short-latency responses and lower-performance long-latency responses. All fish can generate both types of response, but the responses remain unpredictable from trial to trial. We aim to understand how larvae choose to deploy a short- or long-latency response. In earlier work, we found that the short-latency responses are modulated in a similar fashion to startle responses in mammals, in which startle magnitude is inhibited when the startle stimulus is preceded by a weak auditory prepulse. This form of startle modulation, termed prepulse inhibition, is diminished in several neurological conditions, including schizophrenia. Previously, we conducted a screen to identify fish carrying genetic mutations resulting in a reduction in prepulse inhibition. Using these fish, we are performing linkage analysis to map the genetic mutations in the mutants and identify genes required for prepulse inhibition. The experiments will identify circuit components that selectively suppress generation of short-latency responses. In parallel, we are analyzing how long-latency responses are modulated. Brainstem neurons that trigger a motor response must belong to the restricted cohort of neurons that project to the spinal cord. We are therefore sequentially ablating neurons of this class by using a pulsed nitrogen laser and then probing the stimulus threshold and magnitude of the long-latency startle response system. Together, these approaches will allow us to find neuronal mechanisms for the implementation of behavioral choice during startle responses in zebrafish larvae.
Functional mapping of serotonergic neuronal architecture
Transgenic fish mark the dorsal raphe for behavioral analysis of serotonergic neurons
A Gal4 line with selective expression in the dorsal raphe (red) enables dissection of the functional anatomy of the serotonergic system (immunohistochemistry for serotonin: green). Dorsal raphe neurons project broadly to many brain areas, enabling this region to establish behavioral state modulation of sensory-motor reflexes.
The serotonergic system is a major neurotransmitter system modulating behavior in higher vertebrates. To ascertain the role of this system in behavioral control in larval zebrafish, we generated transgenic fish expressing the Gal4 transcription factor in serotonergic neurons. We can thus genetically manipulate these neurons by crossing the fish to lines carrying reporter genes under the control of the UAS promoter, for example to a UAS:nitroreductase line, enabling us to genetically ablate neurons. Consistent with our hypothesis, nitroreductase ablation of serotonergic neurons modulates a discrete subset of behaviors. We have also performed in vivo calcium imaging of serotonergic neurons by injecting the Gal4 transgenic fish with a UAS:GCaMP element, enabling us to show that subsets of dorsal raphe neurons are active in specific behavioral contexts. Finally, we use high-resolution single-neuron tracing by applying mosaic techniques to ascertain specific projection targets of neurons within the raphe. This combination of approaches has permitted us to correlate the connections of single serotonergic neurons with their behavioral role, establishing a neuronal-level functional map of serotonergic anatomy.
Tools for analyzing neuronal circuits that control behavior
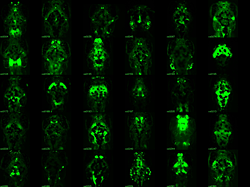
(Click image to enlarge.)
Enhancer trap lines for neurobehavioral research
Examples of transgenic Gal4 enhancer trap lines. Gal4 expression is visualized by crossing lines to a fluorescent UAS:Kaede reporter. All images are dorsal views of the brain, taking advantage of the transparency of larva. Lines with broad expression in the nervous system are recovered along with lines labeling specific structures and lines with very restricted patterns of expression.
The relatively simple nervous system of zebrafish larvae and the larva's restricted range of motor behaviors make it possible to identify neuronal pathways that underlie the entire behavioral repertoire. However, it would be extremely useful to have reporter lines that would permit the manipulation of small groups of neurons known to be involved in a particular motor behavior. We are therefore performing a screen to identify neurons required for normal behavioral performance. To this end, we have created a library of 240 Gal4 enhancer-trap transgenic fish with unique and restricted patterns of neuronal expression. The lines constitute a unique resource for decoding the developmental genetics and neuro-anatomical basis of behavior in zebrafish larvae. We are currently using the lines to ablate the trapped neuronal cohort and screen for defects in a broad range of behaviors, including prepulse inhibition of the startle response. Our work represents the first application of such a screen in a vertebrate organism.
Additional Funding
- Director's Challenge Innovation Award (2011) "Volumetric Imaging of Neuronal Dynamics in the Developing Zebrafish Larva" with Dr. Shroff (NIBIB)
- Japan Society for the Promotion of Science Fellowship to Dr. Yokogawa
Publications
- Fero K, Yokogawa T, Burgess HA. The behavioral repertoire of larval zebrafish. In: Kalueff AV, Cachat JM, eds. Zebrafish Models in Neurobehavioral Research 2011;249-291.
- Burgess HA, Schoch H, Granato M. Distinct retinal pathways drive spatial orientation behaviors in zebrafish navigation. Curr Biol 2010;20:381-386.
- Pei W, Kratz LE, Bernardini I, Sood R, Yokogawa T, Dorward H, Ciccone C, Kelley RI, Anikster Y, Burgess HA, Huizing M, Feldman B. A model of Costeff syndrome reveals metabolic and protective functions of mitochondrial OPA3. Development 2010;137:2587-2596.
Collaborators
- Michael Iadorola, PhD, Laboratory of Sensory Biology, NIDCR, Bethesda, MD
- Fumihito Ono, PhD, Laboratory of Molecular Physiology, NIAAA, Rockville, MD
- Hari Shroff, PhD, Section on High Resolution Optical Imaging, NIBIB, Bethesda, MD
- David Valle, MD, The Johns Hopkins University School of Medicine, Baltimore, MD
Contact
For more information, email haroldburgess@mail.nih.gov or visit ubn.nichd.nih.gov.