You are here: Home > Section on Viral Gene Regulation
Molecular Genetics of Mammalian Retrovirus Replication
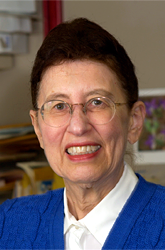
- Judith G. Levin, PhD, Head, Section on Viral Gene Regulation
- Tiyun Wu, PhD, Staff Scientist
- Kamil Hercik, PhD, Postdoctoral Fellow
- Jiyang Jiang, MD, PhD, Postdoctoral Fellow
- Mithun Mitra, PhD, Postdoctoral Fellow
- Klara Post, MS, Senior Research Assistant
- Kathryn S. Hinchee, BS, Postbaccalaureate Fellow
- Gabriel Nam, BA, Postbaccalaureate Fellow
The goal of the research performed in the Section on Viral Gene Regulation is to define the molecular mechanisms responsible for the replication of HIV and related retroviruses and to investigate the role of host proteins that block virus infection. These studies are critical for developing new strategies to combat the AIDS epidemic, which continues to be a global threat to human health. To investigate the individual steps in HIV-1 reverse transcription, a major target of HIV therapy, we have developed reconstituted model systems. Much of our work focuses on the viral nucleocapsid protein (NC), a nucleic acid chaperone that remodels nucleic acid structures so that the most thermodynamically stable conformations are formed—an activity that is critical for highly efficient and specific viral DNA synthesis. We are also investigating the mechanism of antiviral activity of two human cytidine deaminases, APOBEC3G (A3G) and APOBEC3A (A3A). In other studies, our efforts are directed toward understanding the function of the viral capsid protein (CA) in HIV-1 assembly and early postentry events during the course of virus replication in vivo.
Role of nucleocapsid protein in HIV-1 reverse transcription
HIV-1 NC is a small basic protein with two zinc fingers, each containing the invariant CCHC zinc-coordinating residues. Its nucleic acid chaperone function depends on three properties: (i) ability to aggregate nucleic acids, which is important for annealing (N-terminal basic residues); (ii) moderate helix-destabilizing activity (zinc fingers); and (iii) rapid on-off binding kinetics. NC plays a critical role in almost every step of viral DNA synthesis, including minus-strand transfer. In this case, the first product of reverse transcription, (−) strong-stop DNA, is annealed to the RNA sequence at the 3′ end of the genome (acceptor RNA), in a reaction mediated by base-pairing of the complementary repeat regions in the nucleic acid substrates. This is followed by reverse transcriptase (RT)–catalyzed elongation of minus-strand DNA. Current studies focus on comparison of the nucleic acid chaperone activities of HIV-1 NC with HIV-1 Gag and Gag's proteolytic cleavage products. The sequence of Gag in the N to C direction is: matrix (MA)→capsid (CA)→spacer peptide 1 (SP1)→NC→SP2→p6. For this work, we use a reconstituted a minus-strand transfer assay system, an especially sensitive read-out for chaperone function.
We found for the first time that Gag facilitates minus-strand transfer, indicating that it has both annealing and helix-destabilizing activities (1). To compare the activities of Gag and NC in the individual steps in strand transfer, we performed assays of annealing only. These experiments showed that high concentrations of Gag stimulate annealing more strongly than NC, reflecting Gag's 15-fold higher affinity for binding to single-stranded (ss) RNA and ssDNA, as determined by fluorescence anisotropy. In contrast, high concentrations of Gag severely inhibit the DNA extension step, also evident, albeit to a lesser extent, with partially processed Gag proteins, but not with NC. The results are consistent with "nucleic acid-driven multimerization" of Gag (facilitated by CA domain interactions) and the known slow dissociation of Gag from bound nucleic acid, which in turn block RT movement along the single-stranded nucleic acid template. We refer to this phenomenon as the "roadblock" mechanism for inhibition of HIV-1 reverse transcription. Other experiments showed that the critical determinant for the nucleic acid chaperone activity of Gag resides in its NC domain. To evaluate Gag function in a viral setting, we also examined the endogenous RT (ERT) activity of virions expressing Gag or partially processed Gag proteins generated by blocking protease cleavage sites (mutants from Christopher Aiken, Vanderbilt University). Real-time PCR analysis of DNA synthesized in ERT reactions showed that constructs able to release mature NC could undergo minus-strand transfer as efficiently as wild type (WT). Virions expressing uncleaved Gag or a partially cleaved Gag (MA-CA-SP1-NC) have about 29% and 55% of WT activity, respectively. These findings are in accord with the data obtained with the reconstituted system.
Taken together, our results illustrate how the presence of NC in the multi-domain Gag protein modulates the nature of NC's nucleic acid chaperone activity and also help to explain why NC, rather than the Gag precursor, has evolved as the critical cofactor in viral DNA synthesis. The ability of high concentrations of Gag to act as a roadblock to DNA elongation represents a novel regulatory mechanism. More recently, we found that zinc finger (ZF) mutations in the NC domain of Gag, either mutation of CCHC to CCCC in one or both ZFs or deletion of one or both ZFs, do not have a major effect on minus-strand transfer. In fact, differences between the activities of WT Gag and the mutants do not exceed two-fold, in contrast to the very strict requirement for the native ZFs when mature NC is used in the assay. This discrepancy could reflect known differences in the conformations of mature NC and the NC domain in Gag. The roadblock mechanism is also observed with the ZF mutants, but at a two- to four-fold higher protein concentration than is seen with WT Gag. Based on these results, we speculate that the ZF mutations raise the threshold for the roadblock inhibition due to slightly reduced nucleic acid binding affinity and/or possible protein conformational changes. Experiments to determine the effect of Gag and Gag ZF mutations on tRNALys3–primed initiation of (−) strong-stop DNA synthesis are in progress.
In other work, we are continuing to study the RNA removal steps that occur in the course of reverse transcription, and we are now investigating an obligatory reaction preceding minus-strand transfer. During synthesis of (−) strong-stop DNA, the RNase H activity of RT degrades the viral RNA template. As RT reaches the end of the template, short 5′ RNA fragments remain annealed to the DNA because RNase H cleavage of blunt-ended substrates is inefficient. However, these fragments must be removed so that minus-strand transfer can proceed. We hypothesized that fragment removal could be facilitated by NC destabilization of the short duplex and/or RNase H cleavage. To test this hypothesis, we heat-annealed a 20-nt RNA whose sequence is complementary to the 3′ end of (−) strong-stop DNA to the DNA. We added additional components (e.g., acceptor RNA, NC, RT, dNTPs, etc.) and, following incubation, determined the percent transfer product synthesized. Given that strand transfer cannot occur unless the RNA is removed, the assay uses formation of the transfer product as the readout for RNA removal. The results showed that minus-strand transfer occurred to the same extent in the absence or presence of the 20-nt RNA, indicating that the small RNA was removed with high efficiency. In the context of reverse transcription (the context in which RNA removal occurs), both NC and RNase H activity as well as NC's ZF function (ability to coordinate zinc and helix-destabilizing activity) were required for maximal activity. We also measured the efficiency of fragment removal directly, i.e., in the absence of reverse transcription, using gel-shift mobility and FRET assays with radioactively labeled RNA or fluorescently labeled (with FAM) RNA, respectively. NC was able to facilitate RNA removal in each case, and, as in the RT assay, the reaction was dependent on NC's ZF function. These findings are in excellent agreement with our earlier studies of the tRNA removal step in plus-strand transfer (Wu et al., J Virol 1999;73:4794) and the ability of NC to block mispriming during initiation of plus-strand DNA synthesis (Post et al., Nucleic Acids Res 2009;37:1755). Most importantly, we can now conclude that HIV-1 uses a common strategy for all RNA removal reactions that occur during reverse transcription.
Molecular analysis of the functional activities of human APOBEC proteins
Our interest in host proteins that might affect HIV-1 reverse transcription led us to investigate human A3 proteins, a family of seven cytidine deaminases that also have antiviral activity. Our initial studies focused on A3G, a member of the APOBEC protein family, which blocks HIV-1 reverse transcription and replication in the absence of the viral protein known as Vif. In current work, we are studying the human A3A protein, which functions as a cell defense factor by strongly inhibiting retrotransposition of LINE-1 elements, a class of mobile genetic elements. These elements are potentially detrimental to the human genome, as they can insert into coding regions of functional genes. To test the role of A3A deaminase activity in the inhibition of LINE-1 retrotransposition, we performed cell-based assays with A3A mutants and correlated the levels of retrotransposition with catalytic activity. We used structure-guided mutagenesis, based on A3A structural homology to the known structure of the A3G catalytic domain, to design the mutants. In general, we found that mutants defective in deamination were also poor inhibitors of retrotransposition. However, for all A3A mutants tested, the effect of A3A on retrotransposition was dose-dependent, implying that increased A3A cellular expression allows A3A to regain activity against LINE-1, even in the absence of deaminase activity, and suggesting the existence of an additional deaminase-independent mechanism. To obtain further insights into the molecular properties of A3A, we are using highly purified recombinant protein to perform more detailed studies of A3A's cytidine deaminase activity and binding affinity to nucleic acids. We find that A3A is a potent cytidine deaminase and that, with equivalent amounts of enzyme and substrate, deamination is complete within five minutes. A3A binds ssRNA more efficiently than ssDNA, which is surprising, given that ssDNA (and not ssRNA) is the substrate for deaminase activity. In other work, we are collaborating with members of a structural biology group led by Angela Gronenborg, who are determining the three-dimensional structure of A3A by NMR spectroscopy.
Function of HIV-1 capsid protein in virus assembly and early post-entry events
Our laboratory has been investigating the role of the HIV-1 CA protein in early post-entry events, a stage in the infectious process that is still not completely understood. Our initial studies of certain N-terminal domain (NTD) mutants illuminated the intimate connection between infectivity, proper core assembly, structural integrity of the CA protein, and ability to undergo reverse transcription. We are now focusing on the linker region.
Early structural studies indicated that HIV-1 CA consists of two independently folded domains, an NTD (residues 1-145) and a C-terminal domain (CTD) (residues 151-231), which are connected by a short, flexible linker (residues 146-150). Despite advances in our knowledge of CA structure and the considerable sequence conservation of the linker residues, only limited information on their biological and molecular properties has been available until now. To investigate the role of this region in virus assembly and replication, we made alanine-scanning mutations in all of the linker residues (except for P147, which was changed to leucine) and in the two flanking residues (Y145 and L151) (3). We identified three classes of mutants: (i) S146A and T148A, which behave like WT and have infectivity in a single-cycle replication assay; (ii) Y145A, I150A, and L151A, which are noninfectious, assemble unstable cores with aberrant morphology, and synthesize almost no viral DNA; and (iii) P147L and S149A, which display a poorly infectious, attenuated phenotype. Surprisingly, despite their poor replication capacity, infectivity of P147L and S149A env− mutants can be rescued in an efficient and specific manner when these virions are pseudotyped with the vesicular stomatitis virus envelope glycoprotein (VSV-G). Although it is known that VSV-G–mediated entry occurs via pH–dependent endocytosis, why this mode of entry can result in rescue of infectivity is not clear. We have suggested that the known faster fusion kinetics with VSV-G than with HIV-1 Env might result in bypass of the infectivity defect conferred by the P147L and S149A mutations. Several different assays indicated that P147L and S149A cores are unstable. Nevertheless, transmission electron microscopy (TEM) showed that these mutants were able to assemble conical cores (about 50% of the number seen in the WT population) and formed WT-like tubular assemblies from purified CA proteins in vitro. In contrast, Y145A and I150A CA proteins did not assemble into any recognizable structure. The distinctive nature of the P147L and S149A mutants was also apparent in other assays. For example, when viral DNA products were assayed in infected cells by qPCR, both mutants synthesized about 10-fold less DNA than WT, whereas viral DNA products were reduced about 104-fold in cells infected with I150A.
Taken together, our findings demonstrate that the HIV-1 interdomain linker region is a critical determinant of proper core assembly and stability. Moreover, this study has important implications for understanding the molecular nature of HIV-1 assembly, as it underscores the unusual plasticity of CA, which despite the rigorous structural requirements that govern assembly and integrity of viral cores, permits some expression of biological activity even under less than optimal circumstances. Experiments exploiting higher resolution EM techniques are now in progress, in an effort to identify potential ultrastructural differences between the WT and P147L/S149A mutant core structures and the relation to CA function. We have also constructed other mutants for studies that are directed toward isolation of second-site suppressors.
Publications
- Wu T, Datta SAK, Mitra M, Gorelick RJ, Rein A, Levin JG. Fundamental differences between the nucleic acid chaperone activities of HIV-1 nucleocapsid protein and Gag or Gag-derived proteins: biological implications. Virology 2010;405:556-567.
- Levin JG, Mitra M, Mascarenhas A, Musier-Forsyth K. Role of HIV-1 nucleocapsid protein in HIV-1 reverse transcription. RNA Biol 2010;7:754-774.
- Jiang J, Ablan S, Derebail S, Hercík K, Soheilian F, Thomas JA, Tang S, Hewlett I, Nagashima K, Gorelick RJ, Freed EO, Levin JG. The interdomain linker region of HIV-1 capsid protein is a critical determinant of proper core assembly and stability. Virology 2011;421:253-265.
Collaborators
- Eric O. Freed, PhD, HIV Drug Resistance Program, NCI at Frederick, Frederick, MD
- Robert J. Gorelick, PhD, AIDS and Cancer Virus Program, SAIC-Frederick, Inc., NCI at Frederick, Frederick, MD
- Angela M. Gronenborn, PhD, University of Pittsburgh Medical School, Pittsburgh, PA
- Indira Hewlett, PhD, Center for Biologics Evaluation and Research, FDA, Bethesda, MD
- Yasumasa Iwatani, PhD, National Hospital Organization Nagoya Medical Center, Nagoya, Japan
- Karin Musier-Forsyth, PhD, Ohio State University, Columbus, OH
- Alan Rein, PhD, HIV Drug Resistance Program, NCI at Frederick, Frederick, MD
- Ioulia Rouzina, PhD, University of Minnesota, Minneapolis, MN
- Klaus Strebel, PhD, Laboratory of Molecular Microbiology, NIAID, Bethesda, MD
- Shixing Tang, MD, PhD, Center for Biologics Evaluation and Research, FDA, Bethesda, MD
- Mark C. Williams, PhD, Northeastern University, Boston, MA
Contact
For more information, email levinju@mail.nih.gov or visit jlevinlab.nichd.nih.gov.