Building the Zebrafish Lateral Line System
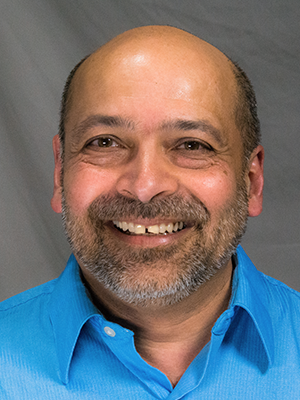
- Ajay Chitnis, MBBS, PhD, Head, Section on Neural Developmental Dynamics
- Damian E. Dalle Nogare, PhD, Staff Scientist
- Gregory Palardy, BS, Research Technician
- Chongmin Wang, MS, Research Technician
- Pritesh Krishnakumar, PhD, Postdoctoral Fellow
- Julia Boehling, BS, Postbaccalaureate Intramural Research Training Award Fellow
- Rachel Dansereau, BS, Postbaccalaureate Intramural Research Training Award Fellow
- Micheal Hilzendeger, BS, Postbaccalaureate Intramural Research Training Award Fellow
- Haille Soderholm, BS, Postbaccalaureate Intramural Research Training Award Fellow
Cells divide, move, adhere, and interact with their neighbors and their environment to determine the formation of multicellular organ systems with unique fates, morphologies, function, and behavior. Our goal is to understand how such interactions determine the self-organization of cell communities in the nervous system of the zebrafish embryo. The lateral line is a mechano-sensory system that helps sense the pattern of water flow over the fish and amphibian body; it consists of sensory organs called neuromasts, which are distributed in a stereotypic pattern over the body surface. Each neuromast has sensory hair cells at its center, surrounded by support cells that serve as progenitors for the production of more hair cells during growth and for the regeneration of neuromasts. The development of this superficial sensory system in zebrafish is spearheaded by the posterior Lateral Line primordia (pLLp), groups of about 150 cells formed on either side of a day-old embryo near the ear. Cells in the primordia migrate collectively under the skin to the tip of the tail, as they divide and reorganize to form nascent neuromasts, which are deposited sequentially from its trailing end. Their journey is easily observed in live transgenic embryos with fluorescent primordium cells. Furthermore, a range of genetic and cellular manipulations can be used to investigate gene function and morphogenesis in the system. Understanding the self-organization of this relatively simple and accessible system in zebrafish will help elucidate the broader principles that determine cell-fate specification, morphogenesis, and collective cell migration in the developing vertebrate nervous system.
NetLogo Agent–based models as tools for understanding the self-organization of cell fate, morphogenesis, and collective migration of the zebrafish pLLp
Understanding how interactions between cells and their environment determine the self-organization of organ systems in a developing embryo is a central goal of developmental biologists. We used a combination of cellular, molecular, and genetic manipulations, coupled with multi-scale live imaging of the zebrafish embryo to understand the early development of the posterior Lateral Line (pLL). The relative simplicity of this sensory system, coupled with the ease with which it can be imaged and manipulated during early zebrafish development, has led to its emergence in recent years as a model for understanding the broader principles of how signaling interactions within and between cells and their environment coordinate cell-fate specification, cell migration, morphogenesis and the self-organization of pattern and form in developing organ systems.
The Lateral Line is a sensory system that has evolved to help fish and amphibians detect the pattern of water flow over their body surface. It consists of sensory organs called neuromasts. Neuromasts have a relatively simple organization and display a characteristic distribution over the body surface. The pLL in zebrafish is a part of this sensory system, with neuromasts distributed on the lateral surface of the trunk and tail along a horizontal stripe that initially runs the length of what corresponds to the horizontal myoseptum. Each neuromast consists of a cluster of cells that come together to form an epithelial rosette. At the center of the rosette are sensory hair cells that extend out of the overlying skin and respond to being bent in a specific direction by water flow. Cells that surround the central sensory hair-cell progenitor are specified as support cells. They provide physical support to central sensory hair cells and also serve as a progenitor population that contributes to specification of additional sensory hair-cell progenitors, which divide to produce additional pairs of sensory hair cells during maturation and regeneration of the neuromasts.
Formation of the pLL system is spearheaded by the pLL primordium, which forms just under the skin as it separates from the pLL placode adjacent to the ear, leaving behind cells of the placode that will form sensory neurons of the pLL ganglion. Cells in the primordium initially have a compact but relatively mesenchymal morphology. However, a subset of the most trailing cells in the primordium begin to acquire distinct apical basal polarity, and they become taller as they assume a more epithelial morphology. These cells come together to form nascent neuromasts, or proto-neuromasts, as they apically constrict and reorganize to form an epithelial rosette around a central cell that is specified as a sensory hair-cell progenitor. After the first proto-neuromast forms at the trailing end, additional proto-neuromasts form sequentially closer to the leading end, as the primordium begins its journey under the skin toward the tip of the tail along the horizontal myoseptum. The most trailing cells in the primordium eventually lose their capacity to migrate, stop moving, and are deposited. Cells that were incorporated into epithelial rosettes are deposited as neuromasts, while surrounding cells that had not been incorporated into the rosettes are deposited individually as inter-neuromast cells between periodically deposited neuromasts. Though proliferation adds cells to the migrating primordium, cell loss owing to deposition from the trailing end exceeds the addition of cells due to proliferation, and the primordium progressively shrinks. Eventually, after depositing 5–6 neuromasts, it ends its journey by forming 2–3 terminal neuromasts near the tip of the tail.
Interactions between primordium cells and their environment determines the self-organization of the zebrafish pLLp as it migrates under the skin from the ear to the tip of the tail, forming and depositing neuromasts to spearhead formation of the pLL sensory system. In a paper published in the past year [Reference 1], we reviewed previously developed models that show how we used the NetLogo agent-based programming environment to visualize and explore how (1) self-generated chemokine gradients determine collective migration, (2) how progressive shrinking of a leading domain dominated by Wnt signaling (regulates cell-fate decisions) can be used to predict patterns of neuromast deposition, and (3) how NetLogo was used as a database for storing and visualizing the results of in toto lineage analysis of all cells in the migrating primordium. We also describe a new model that explores how previously defined interactions between Wnt and Fgf (potent regulators of cell proliferation and differentiation) signaling systems have the potential to determine the periodic formation of center-biased Fgf signaling centers in the wake of a shrinking Wnt system. Together, the models illustrate how the programming environment can be used in diverse ways to integrate what has been learnt from biological experiments about the nature of interactions between cells and their environment, and to explore how such interactions could determine emergent patterns of cell-fate specification, morphogenesis, and collective migration of the zebrafish pLLp.
A sheath of motile cells supports collective migration of the zebrafish pLLp under the skin.
The zebrafish pLLp migrates in a channel formed by the underlying horizontal myoseptum and somites, as well as the overlying skin. While cells in the leading part of the pLLp are flat and have a more mesenchymal morphology, cells in the trailing part progressively reorganize to form the epithelial rosettes called proto-neuromasts. The epithelial cells extend basal cryptic lamellipodia in the direction of migration in response to both chemokine and Fgf signals. We showed that, in addition to the cryptic lamellipodia, the core tall epithelial cells are surrounded by a population of superficial flat motile cells, which extend actin-rich migratory processes apposed to the overlying skin. These thin cells wrap around the deeper proto-neuromasts, forming a continuous sheath of cells around the apical and lateral surface of the pLLp. The processes extended by such cells are highly polarized in the direction of migration, and such directionality, like that of the basal lamellipodia, depends on Fgf signaling. Consistent with interactions of sheath cells with the overlying skin contributing to migration, removal of the skin stalls migration, which is, however, accompanied by some surprising changes: there is a profound change in the morphology of the superficial cells, with directional superficial lamellipodia being replaced by undirected blebs or ruffles. Furthermore, removal of the skin not only affects lamellipodia of superficial cells, it simultaneously alters the morphology and behavior of the basal cryptic lamellipodia, even though the deeper cells that extend them do not directly contact the skin. Directional actin-rich protrusions on both the apical and basal surface and migration are completely and simultaneously restored upon regrowth of the skin over the pLLp. Embedding skin-removed embryos in Matrigel suppresses bleb formation and partially restores formation both superficial and basal lamellipodia. However, only the polarization of the basal lamellipodia is recovered, and the primordium remains unable to migrate robustly. Taken together, such data suggest that the pLLp coordinates collective migration by extending lamellipodia both basally, against the underlying tissue, and superficially, against the skin, and that the presence of the overlying skin is essential for pLLp migration. The manner in which confinement by the skin occurs remains unclear and a question for future studies. However, the suppression of blebs and re-appearance of lamellipodia, following the re-embedding of the skin-removed embryos in Matrigel, which is rich in components of the extracellular matrix (ECM), suggests that re-introduction of ECM components, potential substrates for lamellipodia, are key to their recovery. However, as Matrigel does not lead to recovery of polarized migration, it is likely that the overlying skin contributes in additional ways, including the potential of the skin to confine diffusible signals that serve as directional migratory cues for the primordium and/or the possibility that physical confinement provides mechanical constraints that contribute to migration.
Additional Funding
- DIR Director’s Award FY20–21
Publications
- Dalle Nogare D, Chitnis AB. NetLogo agent-based models as tools for understanding the self-organization of cell fate, morphogenesis and collective migration of the zebrafish posterior Lateral Line primordium. Semin Cell Dev Biol 2020;100:186-198.
- Dalle Nogare D, Natesh, N, Chitnis AB. A sheath of motile cells supports collective migration of the Zebrafish posterior lateral line primordium under the skin. BioRxiv 2019;783043.
Collaborators
- Hari Shroff, PhD, Laboratory of High Resolution Optical Imaging, NIBIB, Bethesda, MD
Contact
For more information, email chitnisa@mail.nih.gov or visit http://chitnislab.nichd.nih.gov.