The Biophysics of Protein-Lipid Interactions in Influenza and Coronavirus, Malaria, and Muscular Dystrophy
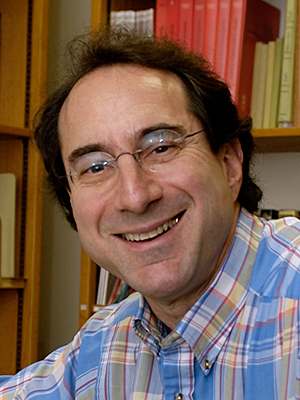
- Joshua Zimmerberg, MD, PhD, Head, Section on Integrative Biophysics
- Paul S. Blank, PhD, Staff Scientist
- Svetlana Glushakova, MD, PhD, Staff Scientist
- Matthias Garten, PhD, Visiting Fellow
- Irene Jimenez Munguia, PhD, Visiting Fellow
- Yuto Kegawa, PhD, Visiting Fellow
- Hang Waters, MS, Biologist
- Jennifer Petersen, PhD, Electron Microscopist
- Elena Mekhedov, MA, Contractor
- Tatyana I. Tenkova-Heuser, PhD, Contractor
- Ludmila Bezrukov, MS, Chemist
- John E. Heuser, MD, Senior Biophysicist
- Glen Humphrey, PhD, Guest Researcher
- Adriana Golding, PhD, Intramural Research Training Award Fellow
- Katherine Chang, BA, Postbaccalaureate Fellow
- Blaise A. Stearns, BS, Postbaccalaureate Fellow
Eukaryotic life must create the many shapes and sizes of the system of internal membranes and organelles that inhabit the variety of cells in nature. Such membranes must remodel in order for cells to repair damaged plasmalemma and deal with infectious agents such as viruses and parasites. Such basic membrane mechanisms must be highly regulated and highly organized in various hierarchies in space and time to allow the organism to thrive despite environmental challenges, genetic instability, unpredictable food supply, and physical trauma. We are using our expertise and the techniques that we perfected over the years to address several different biological problems that have in common the underlying regulation or disturbance of protein/lipid interactions. Thus, the overall goal of this project is to determine the physico-chemical mechanisms of membrane remodeling in cells. This year, we focused on our continued research on the physiology of deadly viruses and parasites by shifting most resources towards the COVID-19 pandemic, in areas closest to our expertise:
- There is great urgency to produce an effective vaccine against COVID-19, not only effective in aging and vulnerable populations, i.e., those more at risk to COVID-19 mortality, but also for early protection from multi-system inflammatory changes in children. Many of the best modern vaccines (e.g., HPV) have been produced using virus-like particles (VLP). Coronavirus presents unique problems in post-translational processing of the Spike (S) protein; our aim is to create a VLP vaccine candidate that recapitulates the native SARS-COV-2 replication cycle of mammalian cellular production and purify pauci-proteinaceous particles (PPP) to acceptable homogeneity for FDA approval as a vaccine candidate.
- Survival of the SARS-COV-2 virus particle for person-to-person transmission and its ability to fuse with the host cell membrane are highly dependent on the viral envelope lipid composition. Determining the lipid composition and physical properties of attenuated virus and VLP will permit the study of membrane stability and fusogenicity under physiological conditions. Our goal is to give the scientific community a VLP to efficiently study virus infection in widely available non-BSL2 (biosafety-level 2) laboratory space.
- Anti-viral therapeutic strategies reducing the impact of the SARS-COV-2 virus should not lag behind vaccine development. The crucial stage of infection is the moment when the viral genome first enters the cytoplasmic space to begin infection, i.e., the fusion of the viral and endosomal membrane of a cell. Merging of two membrane-enclosed compartments is a ubiquitous event in biology and is crucial to fertilization, exocytosis, bone and muscle development, intracellular trafficking, and viral infection. The underlying biophysical determinants in these diverse processes are the same: the fusion and leakage of the mostly phospholipid bilayers that comprise cell membranes. We are working now to incorporate a reporter for the membrane fusion of infection within the COVID-19 VLP described above, which NCATS has agreed to study for antivirals in its medium- and high-throughput screens.
- We are equally committed to a deep understanding of the mechanism by which the Spike proteins of SARS-COV-2, HIV, and influenza work, so as to design drugs against their action. This year, our goal was to model the reason for cooperation between more than one Spike trimer to achieve the fusion of infection.
- In 2018, there were about 228 million cases of malaria worldwide, with about 405,000 malaria deaths. Children aged under five are the most vulnerable group affected by malaria; in 2018, they accounted for 67% (272,000) of global malaria deaths. Our goal in studying malaria is to develop new antimalarial drugs. This year, we defined a new intercellular contact for cells that dwell within other cells: the host-parasite interface (HPI), which may be a drug target.
Exocytotic fusion, compensated by endocytotic fission, are the essence of complex membrane dynamics in living cells. Fusion and fission are key elements of synapses and other dynamic cellular trafficking networks, and are instants when organelles gain or lose their identities. Without exocytosis, the insulin-sensitive glucose transporter would never reach the plasma membrane of a muscle or fat cell, nor would inflammatory cells respond and kill neoplastic or pathogenic invaders, or deal with sick cells. Our Section's earliest work concentrated on model exocytosis systems, the physical properties and theoretical pathways required for exocytosis, and the discovery that tension spreads headgroups for hemifusion, then pulls open fusion pores to allow coalescence of adherent bilayers. However, while enabling us to focus on basic membrane biophysical properties and to help develop a theoretical framework for understanding membrane interactions, model systems are a simplification that ignored the important roles of other cellular components. Last year we reported on lipids that abound in cellular functions known as the phosphoinositides. This year, we focused on the membrane mechanics that govern membrane stability and energetics.
New model for direct physical action of viral fusion proteins
Enveloped viruses include the most dangerous human and animal pathogens, in particular coronavirus, influenza virus, and human immunodeficiency virus (HIV). For these viruses, receptor binding and entry are accomplished by a single viral envelope protein (termed the fusion protein), the structural changes in which trigger the remodeling and merger of the viral and target cellular membranes. The number of fusion proteins required for fusion activity is still under debate, and several studies report this value to range from 1 to 9 for type I fusion proteins. We studied the earliest stage of viral fusion based on the continuum theory of membrane elasticity. We demonstrated that membrane deformations induced by the oblique insertion of amphipathic fusion peptides mediate the lateral interaction of the peptides and drive them to form into a symmetric fusion rosette. The pulling force produced by the structural rearrangements of the fusion protein ectodomains gives additional torque, which deforms the membrane and additionally stabilizes the symmetric fusion rosette, thus allowing a reduction in the number of fusion peptides needed for fusion. The findings can resolve the large range of published cooperativity indices for HIV, influenza, and other type I fusion proteins.
Two possible mechanisms of fusion protein action have been traditionally proposed. One is based on the assumption that the incorporated fusion peptides first of all modify the elastic properties of the target membrane, i.e., its spontaneous curvature. It is thought that the altered spontaneous curvature in the ring-like zone of the fusion rosette might be responsible for the formation of the bulge on the target membrane; the fusing membranes come into close contact at the top of the bulge, which substantially facilitates the merger of membranes. According to the second mechanism, instead of modifying the target membrane, the fusion proteins induce bending torques, causing the formation of bulges and generating pulling forces, thus directly and mechanically bringing two merging membranes into close contact. Based on the results of the present work, we can argue that the second mechanism provides the formation of highly symmetric fusion rosette owing to both tilting of the fusion peptides and pulling them out of the plane of the target membrane, thus ensuring cooperation of the mechanical efforts of fusion proteins. By contrast, the first mechanism uses the symmetry of the fusion rosette to explain the formation of the bulges on fusing membranes, rather than providing an explanation of the symmetry origin. We conclude that the direct mechanical activity of fusion proteins should drive the merger of membranes more reliably than local modification of the elastic properties of the target membrane by the incorporated fusion peptides. This activity could result from the concerted action of different subunits of fusion protein, placing the bound cell receptor molecule as an active player in the scene. The latter assumption could be the answer to the differences in the cooperativity of fusion proteins of influenza and HIV: we would need 39 pH–activated hemagglutinin trimers for effective fusion, while a single receptor-bound gp120/gp41 trimer of HIV would be sufficient.
The interface between the cytoplasm of the protozoan parasite and the cytoplasm of the host cell
For the malaria parasite, the host-parasite Interface (HPI) is an interface between the cytoplasm of the protozoan parasite and the cytoplasm of the human red blood cell. Rather than a simple bag within a bag, the HPI has structural complexity. First, it is formed by two membranes. We discovered that the space between the two membranes varies considerably, such that there are frequent sites of very close membrane contact. The sites of close membrane contact coincide with the location of PfNCR1, a lipid transporter, which allows direct lipid transport from membrane to membrane. The sites of contact exclude EXP2, a nutrient channel. Segregation of the two transporters by the contact sites may be a general mechanism to separate transport systems. We expect that the fundamental conceptual shift, i.e., to consider the HPI as a double membrane, domain-defined structure will generalize to other parasites. Furthermore, the HPI is likely to contain the kind of critical parasite-encoded proteins, such as PfNCR1 and EXP2, against which anti-malarial drugs can be made.
The malaria parasite interfaces with its host erythrocyte (RBC) using a unique organelle, the parasitophorous vacuole (PV). The mechanisms by which its limiting membrane, the parasitophorous vacuolar membrane (PVM), collaborates with the parasite plasma membrane (PPM) to support the transport of proteins, lipids, nutrients, and metabolites between the cytoplasm of the parasite and the cytoplasm of the RBC are obscure. We demonstrated that the structure of the PV is characterized by micrometer-sized regions of especially close apposition between the PVM and the PPM. To determine whether such contact sites are involved in any kind of transport, we localized the PVM nutrient-permeable and protein-export channel EXP2, as well as the PPM lipid transporter PfNCR1. We found that EXP2 is excluded from, but that the PfNCR1 is included within, the regions of close apposition. We conclude that the host-parasite interface is structured to segregate such transporters of hydrophilic and hydrophobic substrates.
Applying the quadratic energy functional monolayer-wise allows a generalization of the linear theory of membrane elasticity.
Living cells are open non-equilibrium systems. To exist, a cell requires precisely controlled maintenance of gradients in the chemical potential between the extracellular environment, the cytoplasm, and the lumen of organelles, of many constituents. The amphiphilic nature of lipid molecules, self-assembling into lipid bilayers, provides an extremely low permeability barrier to both electrolytes and large nonelectrolytes. For the processes of life, a continuous exchange of matter must occur across all membranes. For example, uptake of large molecules and compounds from the outside occurs via endocytosis, phagocytosis, and macro- and micro-pinocytosis, secretion occurs via exocytosis, and intracellular protein trafficking via transport vesicles between endoplasmic reticulum, Golgi apparatus, endosomes, and lysosomes. The movement of such membrane-bound cargo dictates membrane recycling, otherwise cells and organelles would be incapable of maintaining their volumes and shapes. Such ubiquitous and multifarious events, plus the accommodation in lipid bilayers of membrane proteins and transient pores, all require the lipid bilayer to change its topology. Physical models of such topological changes, essential to life, must take into account the energy of membrane deformations within the framework of an adequate theory of elasticity.
The theory of elasticity of lipid membranes is used widely to describe processes of cell membrane remodeling. Classically, the functional of a membranes elastic energy is derived under assumption of small deformations; the membrane is considered as an infinitely thin film. The functional is quadratic on membrane surface curvature, with half of the splay modulus as its proportionality coefficient; it is generally applicable for small deformations only. Any validity of this functional for the regime of strong deformations should be verified experimentally. Recently, research using molecular dynamics simulations challenged the validity of this classic, linear model, i.e., the constancy of the splay modulus for strongly bent membranes. We demonstrated that the quadratic energy functional can still be applied for calculation of the elastic energy of strongly deformed membranes without introducing higher-order terms with additional elastic moduli, but only if applied separately for each lipid monolayer. For cylindrical membranes, both classic and monolayer-wise models yield equally accurate results. For cylindrical deformations we showed experimentally that the elastic energy of lipid monolayers is additive: a low molecular-weight solvent leads to an approximately twofold reduction in the membrane bending stiffness. Accumulation of solvent molecules in the inner monolayer of a membrane cylinder can explain the results, as the solvent partially prevents lipid molecules from splaying there. Thus, the linear theory of elasticity can be expanded through the range of weak to strong deformations. Its simplicity and physical transparency describe various membrane phenomena.
Additional Funding
- NIH Intramural-to-Russia (I-to-R) Program Award
Publications
- Chen CZ, Xu M, Pradhan M, Gorshkov K, Petersen JD, Straus MR, Zhu W, Shinn P, Guo H, Shen M, Klumpp-Thomas C, Michael SG, Zimmerberg J, Zheng W, Whittaker GR. Identifying SARS-CoV-2 entry inhibitors through drug repurposing screens of SARS-S and MERS-S pseudotyped particles. bioRxiv 2020;2020.07.10:197988.
- Garten M, Beck JR, Roth R, Tenkova-Heuser T, Heuser J, Istvan E, Bleck CKE, Goldberg DE, Zimmerberg J. Contacting domains segregate a lipid transporter from a solute transporter in the malarial host-parasite interface. Nat Commun 2020;11:3825.
- Akimov SA, Kondrashov OV, Zimmerberg J, Batishchev OV. Ectodomain pulling combines with fusion peptide inserting to provide cooperative fusion for influenza virus and HIV. Int J Mol Sci 2020;21(15):5411.
- Galimzyanov TR, Bashkirov PV, Blank PS, Zimmerberg J, Batishchev OV, Akimov SA. Monolayerwise application of linear elasticity theory well describes strongly deformed lipid membranes and the effect of solvent. Soft Matter 2020;16:1179–1189.
- Curthoys NM, Mlodzianoski MJ, Parent M, Butler MB, Raut P, Wallace J, Lilieholm J, Mehmood K, Maginnis MS, Waters H, Busse B, Zimmerberg J, Hess ST. Influenza hemagglutinin modulates phosphatidylinositol 4,5-bisphosphate membrane clustering. Biophys J 2019;116:893–909.
Collaborators
- Sergei Akimov, PhD, Frumkin Institute of Physical Chemistry and Electrochemistry, Russian Academy of Sciences, Moscow, Russia
- Pasha Bashkirov, PhD, Frumkin Institute of Physical Chemistry and Electrochemistry, Russian Academy of Sciences, Moscow, Russia
- Oleg Batishchev, PhD, Frumkin Institute of Physical Chemistry and Electrochemistry, Russian Academy of Sciences, Moscow, Russia
- Josh Beck, PhD, Iowa State University, Ames, IA
- Michael J. Blackman, PhD, The Francis Crick Institute and London School of Hygiene & Tropical Medicine, London, United Kingdom
- Christopher Karl Ernst Bleck, PhD, Electron Microscopy Core Facility, NHLBI, Bethesda, MD
- Nikki Curthoys, PhD, University of Maine, Orono, ME
- Andrew Demidowich, MD, PhD, Section on Growth and Obesity, NICHD, Bethesda, MD
- Vadim Frolov, PhD, Universidad del País Vasco, Bilbao, Spain
- Timur Galimzyanov, PhD, Frumkin Institute of Physical Chemistry and Electrochemistry, Russian Academy of Sciences, Moscow, Russia
- Daniel Goldberg, MD, PhD, Washington University St. Louis, St. Louis, MO
- Samuel T. Hess, PhD, University of Maine, Orono, ME
- Ron W. Holz, MD, PhD, University of Michigan Medical School, Ann Arbor, MI
- Mary Kraft, PhD, University of Illinois at Urbana-Champaign, Urbana, IL
- Louis H. Miller, MD, Laboratory of Malaria & Vector Research, NIAID, Bethesda, MD
- Richard Pastor, PhD, Laboratory of Membrane Biophysics, NHLBI, Bethesda, MD
- Thomas S. Reese, MD, Laboratory of Neurobiology, NINDS, Bethesda, MD
- Anna Shnyrova, PhD, Universidad del País Vasco, Bilbao, Spain
- Tobias Spielmann, PhD, Bernhard-Nocht-Institut für Tropenmedizin, Hamburg, Germany
- Peter K. Weber, PhD, Lawrence Livermore National Laboratory, Livermore, CA
- Jack Yanovski, MD, PhD, Section on Growth and Obesity, NICHD, Bethesda, MD
Contact
For more information, email zimmerbj@mail.nih.gov or visit http://irp.nih.gov/pi/joshua-zimmerberg.