Childhood Neurodegenerative Lysosomal Storage Disorders
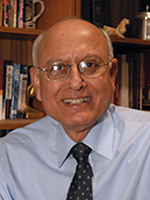
- Anil B. Mukherjee, MD, PhD, Head, Section on Developmental Genetics
- Maria B. Bagh, PhD, Research Fellow
- Abhilash Appu, PhD, Visiting Fellow
- Avisek Mondal, PhD, Visiting Fellow
- Tamal Sadhukhan, PhD, Visiting Fellow
- Sriparna B. Sadhukhan, MSc, Contract Biologist
- Sydney Casey, AB, Postbaccalaureate Intramural Research Training Award Fellow
The Section on Developmental Genetics conducts both basic and translational research into a group of the most common childhood neurodegenerative lysosomal storage disorders (LSDs), called neuronal ceroid lipofuscinoses (NCLs), commonly known as Batten disease. The diseases affect mostly children and there is no curative treatment for any of the NCLs. Mutations in at least 14 different genes (called CLNs) underlie various forms of NCLs. The CLN1, CLN2, CLN5, CLN10, and CLN13 genes encode soluble lysosomal enzymes; The CLN4 and CLN14 encode peripherally associated cytoplasmic proteins; the CLN11 encodes progranulin, a protein in the secretory pathway; and several transmembrane proteins with various subcellular localizations are encoded by CLN3, CLN6, CLN7, CLN8, and CLN12. The infantile NCL (INCL), a devastating neurodegenerative LSD, is caused by inactivating mutations in the CLN1 gene, which encodes a lysosomal depalmitoylating enzyme called palmitoyl-protein thioesterase-1 (PPT1).
Currently, the investigations in our laboratory focus on understanding the molecular mechanisms of pathogenesis underlying INCL (CLN1-disease), juvenile NCL (JNCL: CLN3-disease), and congenital NCL (CNCL: CLN10-disease). Interestingly, all NCL types share some common clinical and pathologic features, such as intracellular accumulation of auto-fluorescent material, epileptic seizures, progressive psychomotor decline resulting predominantly from loss of cortical neurons in the cerebrum, neuro-inflammation, visual impairment resulting from retinal degeneration, and shortened lifespan.
Several years ago, we started investigating the INCL, which is caused by inactivating mutations in the CLN1 gene. Notably, numerous proteins in the body, especially in the brain, undergo a reversible post-translational lipid modification called S-palmitoylation (also called S-acylation), whereby a 16-carbon, saturated fatty acid (generally palmitic acid) is attached to specific cysteine residues in polypeptides via thioester linkage. While S-palmitoylation plays important roles in membrane anchorage of soluble proteins, protein-protein interaction, protein trafficking, and protein stability, these lipid-modified proteins must also be depalmitoylated for recycling or degradation and clearance by lysosomal hydrolases. Thus, dynamic S-palmitoylation (palmitoylation-depalmitoylation), like phosphorylation-dephosphorylation, regulates the function of many proteins, especially in the brain. It requires coordinated action of two types of enzymes with opposing functions. The enzymes that catalyze S-palmitoylation are palmitoyl acyltransferases (PATs), which are zinc-finger proteins with a common DHHC (Asp-His-His-Cys) motif, and they are called ZDHHC PATs or simply ZDHHCs. The mammalian genome encodes a family of 23 ZDHHC PATS.
Similarly, the palmitoyl thioesterases, which depalmitoylate S-acylated proteins, are localized either in the lysosomes like PPT1 or in the cytoplasm like acyl-protein thioesterase-1 (APT1). Recently, a group of protein depalmitoylases called ABHD17 were identified, which catalyze the turnover of N-Ras (a GTP-ase signal-transduction protein).
PPT1 catalyzes the cleavage of thioester linkage of S-palmitoylated proteins, which is vitally important because these lipid-modified proteins are refractory to degradation by lysosomal hydrolases. Thus, PPT1 deficiency leads to lysosomal accumulation of the S-palmitoylated proteins (constituents of ceroid), which has been proposed to be the mechanism of INCL pathogenesis. However, the precise molecular mechanism underlying INCL pathogenesis has remained elusive for more than two decades. Children afflicted with INCL are normal at birth but, by 11 to 18 months of age, exhibit signs of psychomotor retardation. By two years of age, they are completely blind owing to retinal degeneration and, by age four, they manifest no brain activity and remain in a vegetative state for several more years before eventual death. Such grim outcomes underscore the urgent need for the development of rational and effective therapeutic strategies, not only for INCL but also for all NCLs.
The aim of our translational research is to apply the knowledge gained from our basic laboratory investigations to develop novel therapeutic strategies for Batten disease. The results of our earlier investigations on INCL led to a bench-to-bedside clinical trial. Using Cln1-knockout (Cln1–/–) mice, which recapitulate virtually all clinical and pathological features of INCL, we discovered that PPT1 deficiency causes endoplasmic-reticulum (ER) and oxidative stress, which at least in part causes neuronal death by apoptosis. During the past several years, we also delineated a mechanism by which PPT1 deficiency disrupts the recycling of the synaptic vesicle (SV) proteins, which are essential for regenerating fresh SVs to replenish the SV pool size at the nerve terminals to maintain uninterrupted neurotransmission. We also discovered that ER and oxidative stress contribute to neuronal apoptosis and neuro-inflammation in INCL. Further, we found that PPT1 deficiency causes misrouting of the V0a1 subunit of v-ATPase (the proton pump on lysosomal membrane), which dysregulates lysosomal acidification, causing elevated pH and thus adversely affecting lysosomal degradative function.
We also developed a noninvasive method, using MRI and MRS (magnetic resonance spectroscopy), to evaluate the progression of neurodegeneration in Cln1–/– mice. The methods permit repeated evaluation of potential therapeutic agents in treated animals. Application of such methods in our clinical trial with INCL also allowed us to evaluate the progressive decline in brain volume and neurodegeneration. In collaboration with Wadih Zein, we are also conducting studies to determine whether electro-retinography can be used to assess the progressive retinal deterioration in Cln1–/– as well as in Cln1–knock-in (KI) mice, which carry the nonsense mutation in the CLN1 gene commonly found in the INCL patient population in the US. Moreover, we discovered that the blood-brain barrier is disrupted in Cln1–/– mice and that this pathology is ameliorated by treatment with resveratrol, which has antioxidant properties. More recently, we discovered that a nucleophilic small molecule with antioxidant properties, N-(tert-butyl) hydroxylamine (NtBuHA), ameliorates the neurological abnormalities in Cln1–/– mice and extends their lifespan. The compound is currently undergoing preclinical evaluation for the approval of an IND by the FDA. Intriguingly, we discovered that in, Cln1–/– mice, the lysosomes contain insufficient amounts of PPT1-protein and PPT1-enzymatic activity, contributing to neuropathology in this disease. These and related studies provide insight into the complex mechanisms of heritable disorders of neurodegeneration such as CLN1-disease (INCL) as well as CLN3-disease (JNCL) and identify several potential therapeutic targets. Our results suggest that thioesterase-mimetic small molecules such as NtBuHA are potential therapeutics for INCL and may even be for JNCL. More recently, we discovered that cathepsin D (CD) deficiency in lysosomes is a common pathogenic link between CLN1-disease and CLN10-disease (CNCL). Our ongoing laboratory and translational investigations are attempting to advance our knowledge of CLN1, CLN3, and CLN10 diseases. Our long-term plans are to apply our new findings to discover the pathogenic links among various NCLs and to develop novel therapeutic strategies not only for CLN1-disease but also for CLN3 and CLN10-diseases.
Mechanism of dysregulated lysosomal acidification in a mouse model of INCL
In eukaryotic organisms, the lysosome is the primary organelle for intracellular digestion. It contains enzymes that require acidic pH for optimal degradative function. Thus, lysosomal acidification is of fundamental importance in the degradation of macromolecules of intra- and extracellular origin, which are delivered to the lysosome. Moreover, it has been reported that dysregulation of lysosomal acidification contributes to pathogenesis in virtually all LSDs, including NCLs. Furthermore, defective regulation of lysosomal pH has also been reported in common neurodegenerative diseases such as Alzheimer’s and Parkinson’s. However, despite intense studies, the precise mechanism(s) underlying defective lysosomal acidification in these diseases has remained elusive. Lysosomal acidification is regulated by vacuolar ATPase (v-ATPase), a multi-subunit protein complex consisting of the cytosolic V1 sector and the lysosomal membrane-anchored V0-sector. Reversible assembly of V1/V0 sectors on lysosomal membrane maintains functionally active v-ATPase, the proton pump of the cell, which regulates lysosomal acidification.
In contrast to S-palmitoylation, the thioesterases, two of which are cytosolic (APT1 and APT2) and two (PPT1 and PPT2) are localized to the lysosome. Dynamic S-palmitoylation (palmitoylation–depalmitoylation) requires coordinated action of these two groups of enzymes with opposing functions (i.e., ZDHHCs and PPTs), which maintains the steady-state membrane localization and function of numerous important proteins, especially in the brain. By catalyzing depalmitoylation, thioesterases also facilitate recycling or degradation of proteins that undergo S-palmitoylation.
In the present study, we tested a hypothesis that one or more subunits of v-ATPase require S-palmitoylation for endosomal sorting, trafficking and reversible assembly of V1/V0 on lysosomal membrane, which is essential for regulating lysosomal pH, and that Ppt1 deficiency disrupts v-ATPase activity, impairing its proton transport function, thereby dysregulating acidification of lysosomal lumen. Our results show that the lysosomal membrane-anchored V0 sector isoform a1 (V0a1) subunit of v-ATPase indeed undergoes S-palmitoylation, which is required for its sorting and trafficking to the lysosomal membrane. The process appears to be defective in Ppt1–deficient Cln1–/– mice. Notably, we demonstrate that treatment of these mice with a thioesterase (Ppt1)–mimetic small molecule, N-(tert-butyl) hydroxylamine (NtBuHA), restores near-normal v-ATPase activity and rescues the defective lysosomal acidification phenotype. The results demonstrate the potential of NtBuHA as a drug target for INCL.
Palmitoyl-protein thioesterase-1 deficiency dysregulates autophagy in Cln1–/– mice.
Autophagy is an essential cellular degradative process, by which dysfunctional cytoplasmic contents are digested by lysosomal hydrolases. There are several autophagic pathways, which include macroautophagy, microautophagy, and chaperone-mediated autophagy. During autophagy, several vesicle fusion events, including those of autophagosome and lysosome, lead to the eventual degradation and clearance of the cargo within a hybrid structure called the autophagolysosome. Several autophagy genes (called Atg) encoding components of the autophagic process are required for the initiation of autophagy. One of these events is marked by the de novo formation of double-membrane structures called phagophores. Two ubiquitin-like conjugation systems involving the Atg5-Atg12-Atg16 complex and phosphatidylethanolamine-conjugated microtubule-associated protein 1 light chain 3 (LC3-II) are required for the initiation of autophagic process. Emerging evidence indicates that dysregulation of autophagy is one of the central pathogenic mechanisms underlying many human diseases including the LSDs.
Emerging evidence indicates that S-palmitoylation plays critical roles in endosomal sorting and trafficking of subcellular proteins to their destinations, which are especially important in the nervous system. In eukaryotic cells, vesicular transport facilitates intracellular proteins to reach their destinations. In this process, a large superfamily of Ras–like GTPases (called Rabs) play pivotal roles in vesicle formation, cargo selection, sorting, transport, and vesicular fusion, all of which are critical for endocytic and autophagic degradation. One of the proteins belonging to the Rab superfamily of GTPases is Rab7. It directly or indirectly performs several important functions in vesicular trafficking and membrane fusion events that occur between early endosome and late endosome/lysosome. Upon interaction with RILP (Rab–interacting lysosomal protein) on late endosomal/ lysosomal membrane, Rab7, also known as RAB7A, facilitates the autophagosome-lysosome fusion, generating a hybrid organelle called the autolysosome, which facilitates the degradation of cargo from intracellular sources by lysosomal acid hydrolases. Thus, impaired autophagosome-lysosome fusion is one of the suggested mechanisms for the accumulation of un-degraded cargo in the lysosome leading to the pathogenesis of LSDs. In this study, we sought to determine whether autophagy is dysregulated in INCL, and if so, what might be the underlying mechanism(s).
We found that autophagy is dysregulated in Cln1–/– mice and in postmortem brain tissues from an INCL patient, as well as in cultured INCL fibroblasts. Moreover, Rab7 requires S-palmitoylation for trafficking to the late endosomal/lysosomal membrane, which is dysregulated in Cln1–/– mice. Notably, the defect inhibited the Rab7–RILP interaction, which is essential for Rab7 GTPase activity and required for autophagosome-lysosome fusion. The defect impaired degradative functions of the autolysosome (a hybrid structure arising from the fusion of autophagosome with lysosome), causing lysosomal accumulation of un-degraded cargo, and thus leading to INCL pathogenesis. Importantly, treatment of INCL fibroblasts with NtBuHA, a brain-penetrant, PPT1–mimetic small molecule, ameliorated the defective Rab7–RILP interaction. Our findings reveal a previously unrecognized role of CLN1/PPT1 in autophagy and suggest that thioesterase-mimetic small molecules may ameliorate the dysregulated autophagy, with therapeutic implications for INCL.
Dysregulated crosstalk between lysosomal thioesterase PPT1 and cytosolic thioesterase APT1 contributes to neuro-inflammation in INCL mouse model.
Previously, we reported that the thioesterase APT1 undergoes dynamic S-palmitoylation for shuttling between the cytosol and the plasma membrane, where it depalmitoylates H-Ras, regulating its signaling pathway, which stimulates cell proliferation. While we demonstrated that APT1 catalyzes its own depalmitoylation, the ZDHHC(s) that catalyzes its S-palmitoylation had remained unidentified. We found that ZDHHC5 and ZDHHC23 catalyze APT1 S-palmitoylation. Intriguingly, in Cln1–/– mice, deficiency of Ppt1 in the lysosome reduced the levels of ZDHHC5 and ZDHHC23. Remarkably, in the brain of these mice decreased ZDHHC5 and ZDHHC23 levels reduced the levels of S-palmitoylated APT1, thereby increasing the level of plasma membrane-localized H-Ras, which activated its signaling pathway stimulating microglia proliferation. The increase in microglia producing inflammatory mediators contributed to neuro-inflammation, thus leading to neurodegeneration. Our results identified ZDHHC5 and ZDHHC23 as the enzymes that catalyze S-palmitoylation of APT1, and revealed a pathway to microglia proliferation and neuro-inflammation in a lysosomal Ppt1–deficient mouse model of INCL. Our results reveal a previously unrecognized pathway to neurodegeneration in INCL and demonstrate that NtBuHA exerts its neuro-protective effects by suppressing the generation of neurotoxic A1 astrocytes in the brain.
Cln3-mutations, which underlie JNCL, cause significantly reduced levels of Ppt1 protein and Ppt1 enzyme activity in the lysosome.
Given that intracellular accumulation of ceroid is a characteristic of all NCLs, a common pathogenic link for INCL and JNCL has been suggested. It has been reported that CLN3 mutations suppress the exit of the cation-independent mannose 6-phosphate receptor (CI-M6PR) from the trans-Golgi network (TGN). CI-M6PR transports soluble proteins such as PPT1 from the TGN to the lysosome. We therefore hypothesized that CLN3 mutations cause lysosomal PPT1 insufficiency, contributing to JNCL pathogenesis. We found that the lysosomes in Cln3–mutant mice, which mimic JNCL, and those in cultured cells from JNCL patients, contain significantly reduced levels of Ppt1 protein and Ppt1 enzyme activity and progressively accumulate auto-fluorescent ceroid. Furthermore, in JNCL fibroblasts, the V0a1 subunit of v-ATPase is mislocalized to the plasma membrane instead of to its normal location on lysosomal membrane. The defect dysregulates lysosomal acidification, as we previously reported in Cln1–/– mice. Our findings uncover a previously unrecognized role of CLN3 in lysosomal homeostasis and suggest that CLN3 mutations causing lysosomal Ppt1 insufficiency may at least partly contribute to JNCL pathogenesis.
Defective lysosomal Ca++ homeostasis contributes to impaired autophagy in a mouse model of INCL.
Autophagy is dysregulated in virtually all LSDs. Previously, we reported that autophagy is dysregulated in Cln1–/– mice. However, the precise mechanism underlying the defect has remained elusive. We found that, in Cln1–/– mice, lysosomal Ca2+ homeostasis is dysregulated owing to reduced levels of the inositol 1, 4, 5-triphosphate receptor type-1 (IP3R1), which contributes to Ca2+ transport from the ER to the lysosome. Intriguingly, the transcription factor NFATC4, which regulates IP3R1 gene expression, required S-palmitoylation for translocation from the cytosol to the nucleus. Remarkably, the S-palmitoylated NFATC4 level in Cln1–/– mice was substantially reduced, while that of its phosphorylated inactive form was significantly increased in the cytoplasm. The defect suppressed NFATC4 levels in the nucleus, which reduced IP3R1 expression. Consequently, reduced lysosomal Ca2+ level suppressed catalytic activities of Ca2+–dependent lysosomal hydrolases such as cathepsin D and tripeptidyl peptidase-1, which impaired autophagic degradation. Our results reveal a previously unrecognized role of Cln1/Ppt1 in lysosomal Ca2+ homeostasis and suggest that the defect may contribute to impaired autophagy in INCL.
Persistent mTORC1 signaling contributes to neuropathology in INCL mice: amelioration by recombinant PPT1 or Akt1 inhibitor.
Although classically, the lysosome has been considered the terminal organelle for degradation and cellular clearance, emerging evidence indicates that it also relays several nutrient cues to the master growth regulator mTORC1 (mechanistic target of rapamycin complex 1) kinase. Previously, we reported that, in Cln1–/– mice, lysosomal acidification is dysregulated owing to mislocalization of a critical subunit (V0a1) of v-ATPase, which regulates lysosomal acidification. The v-ATPase along with Lamptor1 and SLC38A9 are essential components of the lysosomal nutrient-sensing machinery, which regulates mTORC1 signaling. We found that Lamptor1 and SLC38A9, which are components of the lysosomal nutrient-sensing apparatus, require S-palmitoylation for their localization on the lysosomal membrane and are misrouted in PPT1–deficient Cln1–/– mice. However, despite the disruption of the lysosomal nutrient-sensing machinery, mTORC1 signaling is persistently high in Cln1–/– mouse brains. Notably, in Cln1–/– mice sustained high levels of p-Akt (a serine/threonine-specific protein kinase), p-TSC2 (phospho-tubersclerosis 2), and pPRAS40 (proline-rich Akt substrate of 40 kDa), which promoted the recruitment of Rheb (Ras homolog enriched in brain, a GTP–binding protein) to the lysosomal surface, mediating mTORC1 activation. Remarkably, while mTORC1 signaling in the brain was detectable only on postnatal day 1 of wild-type (WT) mice and was virtually undetectable through adulthood, it was persistently detectable in the brains of Cln1–/– prenatal mice through adulthood, the dysregulated autophagy contributing to neuropathology. Importantly, an Akt1 inhibitor or recombinant PPT1 substantially reduced mTORC1 signaling. Our results reveal a previously unrecognized role of PPT1 in regulating mTORC1 signaling and suggest that inhibitors of Akt1 may have therapeutic implications for INCL.
Publications
- Bagh MB, Peng S, Chandra G, Zhang Z, Singh SP, Pattabiraman N, Liu A, Mukherjee AB. Misrouting of v-ATPase subunit V0a1 dysregulates lysosomal acidification in a neurodegenerative lysosomal storage disease model. Nat Commun 2017;8:8:14612.
- Appu AP, Bagh MB, Sadhukhan T, Mondal A, Casey S, Mukherjee AB. Cln3-mutations underlying juvenile neuronal ceroid lipofuscinosis cause significantly reduced levels of palmitoyl-protein thioesterases-1 (Ppt1)-protein and Ppt1-enzyme activity in the lysosome. J Inherit Metab Dis 2019;42:944-954.
- Sarkar C, Sadhukhan T, Bagh MB, Appu AP, Chandra G, Mondal A, Saha A, Mukherjee AB. Cln1-mutations suppress Rab7-RILP interaction and impair autophagy contributing to neuropathology in a mouse model of INCL. J Inherit Metab Dis 2020;43:1082-1101.
- Mukherjee AB, Appu AP, Sadhukhan T, Casey S, Mondal A, Zhang Z, Bagh MB. Emerging new roles of the lysosome and neuronal ceroid lipofuscinoses. Mol Neurodegener 2019;14(1):4.
Collaborators
- Eva Baker, MD, PhD, Radiology and Imaging Sciences, Clinical Center, NIH, Bethesda, MD
- Yichao Li, MS, Visual Function Core, NEI, Bethesda, MD
- Chris J. McBain, PhD, Section on Cellular and Synaptic Physiology, NICHD, Bethesda, MD
- Kenneth Pelkey, PhD, Section on Cellular and Synaptic Physiology, NICHD, Bethesda, MD
- Haohua Qian, PhD, Visual Function Core, NEI, Bethesda, MD
- Ling-Gang Wu, PhD, Synaptic Transmission Section, NINDS, Bethesda, MD
- Wadih M. Zein, MD, Ophthalmic Genetics and Visual Function Branch, NEI, Bethesda, MD
Contact
For more information, email mukherja@exchange.nih.gov or visit https://www.nichd.nih.gov/research/atNICHD/Investigators/mukherjee.