Modeling the Biophysics of the Membrane
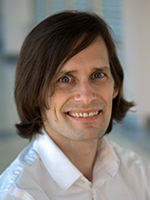
- Alexander J. Sodt, PhD, Head, Unit on Membrane Chemical Physics
- Andrew Beaven, PhD, Postdoctoral Fellow
- Henry H. Lessen, PhD, Postdoctoral Fellow
- Kayla Sapp, PhD, Postdoctoral Fellow
- Victor Chernomordik, PhD, Volunteer
- Mitchell Dorrell, BS, Predoctoral Fellow
- Igor Semyonov, BS, Summer Student
The integrity of lipid membranes is essential for life. They provide spatial separation of the chemical contents of the cell and thus make possible the electrical and chemical potential differences that are used to transmit signals and perform work. However, the membrane must be broken frequently to form, for example, new membrane structures in the cell. The simplest structure is a vesicle to transport cargo. Such vesicles are constantly cycled between organelles and the outer plasma membrane. Thus, there is a careful balance between boundary-establishing membrane fidelity and the necessary ability of the cell to change these boundaries.
The challenge in studying the membrane is its complexity. The membrane is a thin sheet of small molecules, i.e., lipids. There are hundreds of types of lipids in the cell. Each lipid changes the properties of the membrane in its vicinity, sometimes making the sheet stiffer, sometimes softer, and sometimes acting to bend the membrane into a ball or tube. Furthermore, the lipids are constantly jostling and tangling with both each other and with proteins embedded in the membrane. To predict of how membranes are reshaped thus requires not only knowing how lipids affect the properties of the membrane surface, but also the location of specific lipids.
The question as to how molecular scale features influence extensive biological processes must be answered in the language of physical laws. Physics is the language of mechanism at the molecular scale. The challenge is linking physics to the “big” processes that happen in life. Our lab uses detailed physics-driven molecular simulation to ‘build up’ models that can be applied at the much larger level of the cell, which requires retaining important information and eliminating irrelevant details. The software our lab develops is based on the models that we are building. Thus, a broad objective of our research is to create a publicly available software package that can be used either as a stand-alone application for analyzing membrane-reshaping processes or as a library for cellular-scale modeling packages for which the role of the membrane may be unclear or unanticipated.
Another key component of our research is to seek the best possible validation of our models. Few techniques are able to yield molecular information about lipids. Recent breakthroughs that break the diffraction-limit barrier are typically only applicable to static structures much larger than a molecular dye. In contrast, lipids are small and dynamic. We briefly discuss below our breakthroughs for comparing neutron scattering with our molecular simulations of complex lipid bilayers.
The projects use the NIH Biowulf computing cluster to run simulations and models. We use molecular dynamics software (such as NAMD and CHARMM) to conduct molecular simulations. In-house software development for public distribution is a key element of the lab's work.
Determining the structure of complex membranes with neutron scattering and molecular simulation
The structure of molecular bilayers is challenging to characterize owing to its dynamic nature. Structural biological techniques can resolve the atomic positions of proteins because a protein is represented well by a single or limited number of structures. In contrast, biological membranes are highly dynamic, meaning both that the surface as a whole undulates (like the surface of a lake) and that the many constituent lipids rearrange rapidly into different patterns determined by their chemistry. The patterns are undetectable by the methodology used to characterize proteins.
Neutrons offers exciting advantages over light microscopy. Unlike a fluorophore (or dye) that absorbs and emits (inelastically) photons from its diffuse electronic cloud, neutrons interact elastically with the atomic nuclei of lipids. That is, if one shoots a beam of neutrons at a sample, they bounce off the atoms of the sample, changing direction but not speed. At this quantum length-scale, they generate a specific pattern at the detector of a neutron experiment. While this so-called interference pattern reflects that complex patterning of lipids on a surface, it is nearly impossible to directly reconstruct into the original shape and patterning of lipids. With our new developments, scientists can use molecular simulations as a practical bridge between signal and structure.
In two studies we published this year, we develop new approaches that enable molecular simulations to capture the scattering from experimental samples with complex lipid compositions. The first, in collaboration with Fred Heberle, Edward Lyman, and John Katsaras, computes “lateral” scattering from bilayers. The majority of scattering from a bilayer reflects its shape as a thin sheet; this characteristic signal has been previously well characterized. A much smaller component of the scattering is a result of the in-plane lateral distributions of lipids. Understanding the component is critically important for determining how lipid chemistry leads to rearrangement within the surface, and eventually what shapes a bilayer will form. Developing this method, along with the accompanying software, required significant mathematical developments, as well as an extensive validation test [Reference 1].
We also modelled the scattering from large, highly curved bilayer structures, such as vesicles. The methodology [Reference 2] combines our continuum and molecular simulations to create a complete picture of scattering from complex objects. Essentially, one can now conduct a relatively short simulation of a complex lipid bilayer followed by a computationally modest continuum model of a large shape (too large for a molecular simulation), and compute the full neutron scattering.
Publications
- Dorrell M, Beaven AH, Sodt AJ. A combined molecular/continuum-modeling approach to predict the small-angle neutron scattering of curved membranes. Chem Phys Lipids 2020;233:104983.
- Dorrell MW, Heberle FA, Katsaras J, Maibaum L, Lyman E, Sodt AJ. Laterally resolved small-angle scattering intensity from lipid bilayer simulations: an exact and a limited-range treatment. J Chem Theory Comput 2020;16:5287-5300.
Collaborators
- Frederick Heberle, PhD, Oak Ridge National Laboratory, Oak Ridge, TN
- John Katsaras, PhD, Oak Ridge National Laboratory, Oak Ridge, TN
- Edward Lyman, PhD, University of Delaware, Newark, DE
- Lutz Maibaum, PhD, University of Washington, Seattle, WA
Contact
For more information, email alexander.sodt@nih.gov or visit http://sodtlab.nichd.nih.gov.