Molecular Genetics of Heritable Human Disorders
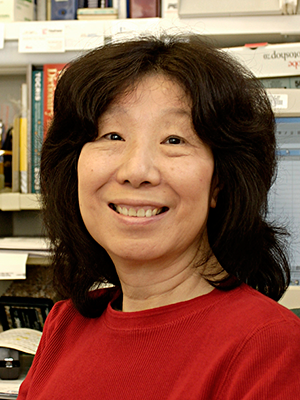
- Janice Y. Chou, PhD, Head, Section on Cellular Differentiation
- Irina Arnaoutova, PhD, Staff Scientist
- Lisa Zhang, PhD, Staff Scientist
- Sudeep Gautam, PhD, Visiting Fellow
- Nelson George, PhD, Visiting Fellow
- Hung-Dar Chen, PhD, Contract Technician
- Cheol Lee, PhD, Contract Technician
We conduct research to delineate the pathophysiology of and develop novel therapies for the glycogen storage disease type I (GSD-I) subtypes GSD-Ia and GSD-Ib. GSD-Ia is caused by a deficiency in the liver/kidney/intestine–restricted glucose-6-phosphatase-α (G6Pase-α or G6PC), and GSD-Ib is caused by a deficiency in the ubiquitously expressed glucose-6-phosphate transporter (G6PT or SLC37A4). G6Pase-α is an endoplasmic-reticulum (ER) transmembrane protein that regulates intracellular glucose production by catalyzing the hydrolysis of G6P to glucose and phosphate. The active site of G6Pase-α faces into the ER lumen and depends on G6PT, another ER transmembrane protein, to translocate G6P from the cytoplasm into the ER lumen. To function, G6Pase-α couples with G6PT to form a functional G6Pase-α/G6PT complex, which maintains interprandial glucose homeostasis. GSD-Ia and GSD-Ib patients manifest a common metabolic phenotype of impaired glucose homeostasis and long-term complications of hepatocellular adenoma/carcinoma (HCA/HCC) and renal disease. There is no cure for GSD-Ia and GSD-Ib. The current dietary therapies have enabled GSD-I patients to maintain a normalized metabolic phenotype if strictly adhered to. However, the underlying pathological processes remain uncorrected, and HCA/HCC and renal disease still occur in metabolically compensated GSD-I patients. We generated animal models of GSD-Ia and GSD-Ib, which are being exploited to both delineate the disease more precisely and develop new treatment approaches, including gene therapy. We also generated G6PC– and G6PT–expressing recombinant adeno-associated virus (rAAV) vectors and showed that rAAV vector–mediated gene therapy for GSD-Ia and GSD-Ib is safe and efficacious. Our rAAV–G6PC/rAAV-coG6PC vector (US patent #9,644,216) technology was licensed to Ultragenyx Pharmaceutical Inc. (Novato, CA), who launched a phase I/II clinical trial (NCT03517085) in 2018, followed by a phase III clinical trial (NCT05139316) in 2022, using the rAAV-GPE–coG6PC vector. To explore alternative genetic technologies for GSD-I therapies, we have established formal collaborations under the CRADA with CRISPR Therapeutics (Boston, MA) and Beam Therapeutics (Boston, MA) to evaluate the efficacy of CRISPR/Cas9–based and adenine base editor (ABE)–based gene editing systems, respectively, to correct gene-specific G6PC mutations in animal models of GSD-Ia.
Figure 1.
Molecular mechanism underlying hepatic autophagy impairment in GSD-Ib
Correction of metabolic abnormalities in a mouse model of glycogen storage disease type Ia by CRISPR/Cas9–based gene editing
The rAAV-coG6PC vector used in the current phase III clinical trial is episomally expressed, and the long-term durability of expression in humans is currently being established. We therefore sought to explore the use of the CRISPR/Cas9 technology to correct a pathogenic GSD-Ia variant in its native genetic locus. The most prevalent pathogenic mutation identified in Caucasian GSD-Ia patients is G6PC-p.R83C, representing 32% of diseased alleles. Using the CRISPR/Cas9–based gene editing technology, we generated a GSD-Ia mouse disease model, the G6pc-R83C mouse homozygous for the G6PC-p.R83C mutation and showed that the G6pc-R83C mice manifest impaired glucose homeostasis mimicking that of human GSD-Ia. We then used a CRISPR/Cas9–based gene-editing system to treat newborn G6pc-R83C mice and showed that the treated mice grew normally to age 16 weeks without hypoglycemia seizures. The treated G6pc-R83C mice, expressing 3% or more of normal hepatic G6Pase-α activity, maintained glucose homeostasis, displayed normalized blood metabolites, and could sustain 24 hours of fasting. Taken together, we have developed a second-generation therapy in which in vivo correction of a pathogenic G6PC-p.R83C variant in its native genetic locus could lead to potentially permanent, durable, long-term correction of the GSD-Ia disorder [Reference 1].
Molecular mechanism underlying hepatic autophagy impairment in GSD-Ib
Clinically, GSD-Ib patients manifest a metabolic phenotype of impaired blood glucose homeostasis and a long-term risk of hepatocellular adenoma/carcinoma (HCA/HCC). The etiology of HCA/HCC in GSD-Ib is unknown. Studies have shown that deficiency in autophagy, an evolutionarily conserved, degradative process that produces energy and building blocks through lysosomal degradation of intracellular proteins and organelles in times of nutrient deprivation and environmental stresses, contributes to hepatocarcinogenesis. Autophagy can be regulated positively by the deacetylase sirtuin 1 (SIRT1), AMP–activated protein kinase (AMPK), and forkhead box O (FoxO) transcription factor family members. In the liver, AMPK is activated via phosphorylation of the AMPK α-subunit at residue T172 by the liver kinase B-1 (LKB1), a serine/threonine kinase.
To understand the pathways contributing to hepatocarcinogenesis in GSD-Ib, we hypothesized that impaired hepatic autophagy is a significant contributor. We showed that G6PT deficiency leads to impaired hepatic autophagy, evident from attenuated expression of many components of the autophagy network, reduced autophagosome formation, and reduced autophagy flux. The G6PT–deficient liver displayed impaired SIRT1 and AMPK signaling, along with reduced expression of SIRT1, FoxO3a, LKB1, and the active p-AMPK. Importantly, we showed that overexpression of either SIRT1 or LKB1 in G6PT–deficient liver restored autophagy and SIRT1/FoxO3a and LKB1/AMPK signaling. The hepatosteatosis in G6PT–deficient liver reduced SIRT1 expression. LKB1 overexpression reduced hepatic triglycerides levels, providing a potential link between LKB1/AMPK signaling upregulation and the increase in SIRT1 expression. In conclusion, downregulation of SIRT1/FoxO3a and LKB1/AMPK signaling underlies impaired hepatic autophagy, which may contribute to HCA/HCC development in GSD-Ib. Understanding this mechanism may guide future therapies [Reference 2].
Gene therapy for GSD-Ia
We generated four efficacious G6PC gene transfer rAAV vectors for GSD-Ia gene therapy: rAAV-G6PC expressing the wild-type (WT) G6PC, rAAV-coG6PC expressing a codon-optimized (co) G6PC, rAAV-G6PC-S298C expressing a G6PC-S298C variant with increased efficacy, and rAAV-coG6PC-S298C. As stated above, our rAAV–G6PC/rAAV-coG6PC vector (US patent #9,644,216) technology was licensed to Ultragenyx Pharmaceutical Inc., who launched a phase I/II clinical trial (NCT03517085) in 2018, followed by a phase III clinical trial (NCT05139316) in 2022 using the rAAV-GPE-coG6PC vector.
To examine the long-term efficacy of such rAAV vectors, we conducted a long-term (66–76 week) gene transfer study in G6pc–/– mice using these rAAV vectors. All treated G6pc–/– mice survived to age 66-76 weeks, and the outcomes were additive. Hepatic G6Pase-α activities in rAAV-G6PC-S298C–, rAAV-coG6PC–, and rAAV-coG6PC-S298C–treated G6pc–/– mice were 1.7-, 1.7-, and 4.4–fold higher, respectively, than that in rAAV-G6PC-WT–treated mice. The efficacy of the rAAV-coG6PC-S298C vector is 2.6–fold higher than the rAAV-coG6PC vector currently used in phase III clinical trial (NCT05139316). Taken together, the rAAV-G6PC-S298C and rAAV-coG6PC-S298C vectors offer attractive clinical alternatives [Reference 3].
Evaluation of the adenine base editor–based gene-editing system to correct a pathogenic G6PC mutation in a humanized mouse model of GSD-Ia
We explored the adenine base editor (ABE)–based technologies that enable a programmable conversion of A•T to G•C in genomic DNA for GSD-Ia therapies. The ABE system works in both dividing and non-dividing cells, is reported to produce virtually no indels or off-target editing in the genome, and can correct a pathogenic variant in its native genetic locus, leading to permanent, therapeutically effective long-term expression. This is a collaborative study with Beam Therapeutics, Cambridge, MA under a CRADA.
The G6PC-p.R83C is the most prevalent pathogenic mutation identified in Caucasian GSD-Ia patients that contains a single G→A transition in the G6PC gene. We first generated a homozygous humanized R83C/R83C mouse strain, the G6PC-R83C mouse, by inserting the entire coding sequence of the human G6PC-p.R83C along with human G6PC 3′ UTR into exon 1 of the mouse G6pc gene at the ATG start codon. The insertion places the human transcript under the control of the native mouse G6pc promoter/enhancer. The mouse G6pc gene is disrupted by a premature STOP codon created in the mouse G6pc exon 1. We showed that the G6PC-R83C mice manifest impaired glucose homeostasis characterized by growth retardation, hypoglycemia, hyperlipidemia, hyperuricemia, hepatomegaly, and nephromegaly, mimicking the abnormal metabolic phenotype of human GSD-Ia. We then treated newborn G6PC-R83C mice with lipid nanoparticles encompassing the guide RNA and mRNA encoding ABE (LNP-ABE) and showed that the treated mice grew normally to age eight weeks without hypoglycemia seizures. The LNP-ABE–treated G6PC-R83C mice expressed significant levels of hepatic G6Pase-α activity with an editing efficiency of up to about 60% and displayed normalized blood metabolite profiles and could tolerate 24 hours of fasting. Taken together, our data demonstrate the potential of base-editing to correct the G6PC-p.R83C mutation in its native genetic locus, which could lead to potentially permanent, durable, long-term correction of the GSD-Ia disorder.
Targeting Wnt/β-catenin signaling reduces renal fibrosis in murine GSD-Ia.
GSD-Ia patients manifest nephromegaly caused by marked glycogen accumulation and nephropathy. The current dietary therapies have significantly alleviated metabolic abnormalities and delayed chronic renal disease and renal insufficiency in GSD-Ia patients. However, the underlying pathological processes remain uncorrected: glomerular hyperfiltration, hypercalciuria, hypocitraturia and urinary albumin excretion still occur in metabolically compensated GSD-Ia patients. We showed that one mechanism underlying GSD-Ia nephropathy is fibrosis mediated by activation of the renin-angiotensin system (RAS). The Wnt/β-catenin signaling that promotes fibrosis controls the expression of RAS genes. We hypothesized that elevated renal glycogen could elicit acute kidney injury (AKI) by activating Wnt/β-catenin signaling and promoting fibrosis. We showed that G6pc–/– mice displayed impaired renal glucose homeostasis and AKI. Renal levels of β-catenin increased markedly in G6pc–/– mice during postnatal development, along with elevated renal levels of renin, angiotensinogen, and the transcription factor snail1. Renal fibrosis was evident by elevated renal levels of α-smooth muscle actin (α-SMA) and extracellular matrix (ECM) proteins. ICG-001, a β-catenin inhibitor, reduced renal levels of active β-catenin, renin, snail1, and α-SMA, indicating that targeting the Wnt/β-catenin signaling is a promising therapeutic strategy for GSD-Ia nephropathy.
Additional Funding
- The Children’s Fund for Glycogen Storage Disease Research
- CRISPR Therapeutics (Cambridge, MA), under a cooperative research and development agreement (CRADA)
- Beam Therapeutics, Inc (Cambridge, MA), under a CRADA
Publications
- Arnaoutova I, Zhang L, Chen HD, Mansfield BC, Chou JY. Correction of metabolic abnormalities in a mouse model of glycogen storage disease type Ia by CRISPR/Cas9-based gene editing. Mol Ther 2021 29(4):1602–1610.
- Gautam S, Zhang L, Lee C, Arnaoutova I, Chen HD, Resaz R, Eva A, Mansfield BC, Chou JY. Molecular mechanism underlying hepatic autophagy impairment in glycogen storage disease type Ib. Hum Mol Genet 2022 online ahead of print.
- Zhang L, Lee C, Arnaoutova I, Anduaga J, Starost MF, Mansfield BC, Chou JY. Gene therapy using a novel G6PC-S298C variant enhances the long-term efficacy for treating glycogen storage disease type Ia. Biochem Biophys Res Commun 2020 527:824–830.
Collaborators
- Alessandra Eva, PhD, Istituto Giannina Gaslini, Genoa, Italy
- Brian C. Mansfield, PhD, Foundation Fighting Blindness, Columbia, MD
- Matthew F. Starost, PhD, Diagnostic & Research Services Branch, Division of Veterinary Resources, NIH, Bethesda, MD
- David A. Weinstein, MD, MSc, University of Connecticut School of Medicine, Farmington, CT
Contact
For more information, email chou@helix.nih.gov or visit https://www.nichd.nih.gov/research/atNICHD/Investigators/chou.