Studies on DNA Replication, Repair, and Mutagenesis in Eukaryotic and Prokaryotic Cells
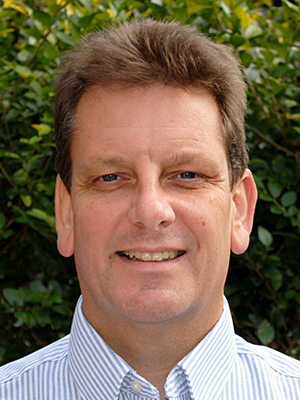
- Roger Woodgate, PhD, Chief, Section on DNA Replication, Repair, and Mutagenesis
- John P. McDonald, PhD, Biologist
- Mary McLenigan, BS, Chemist
- Nicholas W. Ashton, PhD, Postdoctoral Visiting Fellow
- Marcella T. Latancia, PhD, Postdoctoral Visiting Fellow
- Natalia C. Moreno, PhD, Postdoctoral Visiting Fellow
- Mallory R. Smith, PhD, Postdoctoral Intramural Research Training Award Fellow
- Dana A. D'Orlando, BS, Postbaccalaureate Intramural Research Training Award Fellow
- Nickolas G. Moeckel, BS, Postbaccalaureate Intramural Research Training Award Fellow
Under optimal conditions, the fidelity of DNA replication is extremely high. Indeed, it is estimated that, on average, only one error occurs for every 10 billion bases replicated. However, given that living organisms are continually subjected to a variety of endogenous and exogenous DNA–damaging agents, optimal conditions rarely prevail in vivo. While all organisms have evolved elaborate repair pathways to deal with such damage, the pathways rarely operate with 100% efficiency. Thus, persisting DNA lesions are replicated, but with much lower fidelity than in undamaged DNA. Our aim is to understand the molecular mechanisms by which mutations are introduced into damaged DNA. The process, commonly referred to as trans-lesion DNA synthesis (TLS), is facilitated by one or more members of the Y-family of DNA polymerases, which are conserved from bacteria to humans. Based on phylogenetic relationships, Y-family polymerases may be broadly classified into five subfamilies: DinB–like (pol IV/pol kappa–like) proteins are ubiquitous and found in all domains of life; in contrast, the Rev1–like, Rad30A (pol eta)–like, and Rad30B (pol iota)–like polymerases are found only in eukaryotes; and the UmuC (polV)–like polymerases only in prokaryotes. We continue to investigate TLS in all three domains of life: bacteria, archaea, and eukaryotes.
Prokaryotic studies
Pathogenic bacteria pose a major global threat through the precipitous emergence of multidrug resistant strains. Horizontal transfer of mobile genetic elements, including R-plasmids, integrative-conjugative elements (ICEs) and chromosomal instabilities, accompanied by high mutation rates, are among the key factors driving antibiotic resistance. Recent findings have provided new insights into sources of mutagenesis leading to drug resistance. Elevated mutagenesis accompanying the induction of the SOS stress response caused by exposure to antibiotics and, more generally, to a wide variety of exogeneous DNA damage, have been linked to the development of bacterial antibiotic resistance. For example, exposure of clinical isolates of E. coli to ciprofloxacin or zidovudine and Acinetobacter baumannii to UV or the alkylating agent methyl methanesulfonate (MMS) resulted in the development of antibiotic resistance. There is a paucity of data documenting specific contributions of SOS–induced proteins, including pathogenic bacterial homologs of the E. coli LexA repressor RecA, and low-fidelity DNA polymerase V (pol V), toward the acquisition of drug resistance. Homologs of pol V have been identified in a variety of Gammaproteobacteria, many of which are pathogenic. In addition to homologs encoded chromosomally, many pol V homologs are found on mobile genetic elements that can be horizontally transferred between different bacterial species. We referred to generic pol V homologs encoded on the chromosomes of pathogens as Pathogen Encoded Pols, abbreviated as PEPols. Homologous enzymes encoded by mobile elements, particularly integrative conjugative elements (ICEs), were referred to as Mobile Element encoded Pols (MEPols).
In collaboration with Myron Goodman’s laboratory, we used Rum pol (RumA′2B), from the integrative conjugative element (ICE) R391, as a model mobile element–encoded polymerase (MEPol). The highly mutagenic Rum pol is transferred horizontally into a variety of recipient cells, including many pathogens. Moving between species, it is unclear whether Rum pol can function on its own or requires activation by host factors. To test this hypothesis, we investigated the biochemical and in vivo mutagenic behavior of Rum Mut assembled with RecA homologs purified from seven bacterial species; four clinical bacterial isolates in which the rumAB encoding R391/SXT family of ICE’s have been previously identified (E. coli, V. cholerae, P. rettgeri, K. pneuomniae), and from three clinical isolates lacking rumAB genes (P. aeruginosa, M. tuberculosis, S. aureus).
First, we demonstrated that Rum pol biochemical activity requires the formation of a physical mutasomal complex, Rum Mut, containing RumA′2B-RecA-ATP, with RecA being donated by each recipient bacteria. Interestingly, Rum Mut–specific activities in vitro and mutagenesis rates in vivo depended on the phylogenetic distance of host-cell RecA from E. coli RecA. We hypothesized that Rum pol, which is a highly conserved and effective mobile catalyst of rapid evolution, has the potential to generate a broad mutational landscape that could serve to ensure bacterial adaptation in antibiotic-rich environments, leading to the establishment of antibiotic resistance.
Eukaryotic studies
The Y-family DNA polymerases are responsible for copying damaged DNA during DNA replication in a process called translesion synthesis (TLS). These enzymes are highly specialized in order to accommodate different structural DNA distortions caused by a wide variety of DNA lesions. The Y-family is divided into six phylogenetically distinct subfamilies: two UmuC (pol V) branches; Rad30A/POLH (pol eta); Rad30B/POLI (pol iota); DinB (pol IV, Dpo4, pol kappa); and Rev. Across the different domains of life, Y-family polymerase subfamilies are found in various combinations. For example, UmuC orthologs are only detected in Gram-positive and Gram-negative bacteria, whereas Rev1 and Rad30A/B orthologs are only detected in eukaryotes. The DinB subfamily is the most evolutionarily conserved, with members scattered throughout all three domains of life, from unicellular bacteria to humans. However, differences in the distribution of Y-family DNA pols are present within each kingdom. For example, the eukaryote Saccharomyces cerevisiae contains neither a polk nor a poli gene. Indeed, it was originally assumed that poli was expressed only in higher eukaryotes. However, next -generation whole-genome sequencing revealed that pol iota orthologs are actually distributed throughout the whole Eukaryota domain. One example is the thermophilic fungus Thermomyces lanuginosus, which possesses all four eukaryotic Y-family subfamilies, much like humans, in contrast to its fungal relatives S. cerevisiae and Schizosaccharomyces pombe. We wished to determine whether there is a logic in a such seemingly random distribution of pol iota. Using phylogenetic analysis and comparing the biochemical characterization of Y-family pols from different species, we hoped to shed some light on this question.
To do so, we described the identification, purification, and characterization of thermostable eukaryotic orthologs of pol eta, pol iota, pol kappa, and Rev1 from T. lanuginosus. Biochemical characterization of TLS DNA pols eta, iota, kappa, and Rev1 included determination of the enzyme’s fidelity, processivity, thermostability, metal ion requirements, and TLS specificity during bypass of cyclobutane pyrimidine dimers (CPDs), abasic sites, and benzo[a]pyrene diol epoxide (BPDE) adducts. Our findings serve as a basis for comparative analysis of the properties of proteins from different species and provided an important insight into the functional evolution of the Y-family polymerases.
Apart from the expected increased thermostability of the T. lanuginosus Y-family pols, their major biochemical properties are very similar to properties of their human counterparts. In particular, both Rad30B homologs (T. lanuginosus and human pol iota) exhibit remarkably low fidelity during DNA synthesis that is template sequence–dependent. It was previously hypothesized that higher organisms had acquired this property during eukaryotic evolution, but these observations imply that poli originated earlier than previously known, suggesting a critical cellular function in both lower and higher eukaryotes.
Publications
- Vaisman A, McDonald JP, Smith MR, Aspelund SL, Evans TC Jr, Woodgate R. Identification and characterization of thermostable Y-Family DNA polymerases η, ι, κ and Rev1 from a lower eukaryote, Thermomyces lanuginosus. Front Mol Biosci 2021 8:778400.
- Ojha D, Jaszczur MM, Sikand A, McDonald JP, Robinson A, van Oijen AM, Mak CH, Pinaud F, Cox MM, Woodgate R, Goodman MF. Host cell RecA activates a mobile element-encoded mutagenic DNA polymerase. Nucleic Acids Res 2022 50:6854–6859.
Collaborators
- Irina Bezsonova, PhD, University of Connecticut, Farmington, CT
- Anders R. Clausen, PhD, Göteborgs Universitet, Göteborg, Sweden
- Michael Cox, PhD, University of Wisconsin, Madison, WI
- Tom C. Evans, PhD, New England Biolabs, Ipswich, MA
- Iwona Fijlakowska, PhD, Polish Academy of Sciences, Warsaw, Poland
- Martín Gonzalez, PhD, Southwestern University, Georgetown, TX
- Myron F. Goodman, PhD, University of Southern California, Los Angeles, CA
- Karolina Makiela-Dzbenska, PhD, Polish Academy of Sciences, Warsaw, Poland
- Justyna McIntyre, PhD, Polish Academy of Sciences, Warsaw, Poland
- Andrew Robinson, PhD, University of Wollongong, Wollongong, Australia
- Ewa Sledziewska-Gojska, PhD, Polish Academy of Sciences, Warsaw, Poland
- Antoine Van Oijen, PhD, University of Wollongong, Wollongong, Australia
- Digby Warner, PhD, University of Cape Town, Cape Town, South Africa
- Wei Yang, PhD, Laboratory of Molecular Biology, NIDDK, Bethesda, MD
Contact
For more information, email woodgate@.nih.gov or visit https://sdrrm.nichd.nih.gov.