Modeling the Biophysics of Cellular Membranes
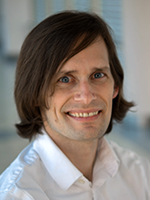
- Alexander J. Sodt, PhD, Head, Unit on Membrane Chemical Physics
- Andrew Beaven, PhD, Postdoctoral Fellow
- Amirali Hossein, PhD, Postdoctoral Fellow
- Henry H. Lessen, PhD, Postdoctoral Fellow
- Kayla Sapp, PhD, Postdoctoral Fellow
The integrity of lipid membranes is essential for life. They provide spatial separation of the chemical contents of the cell and thus make possible the electrical and chemical potential differences that are used to transmit signals and perform work. However, the membrane must be broken frequently to form, for example, new membrane structures in the cell. The simplest structure is a vesicle to transport cargo. Such vesicles are constantly cycled between organelles and the outer plasma membrane. Thus, there is a careful balance between boundary-establishing membrane fidelity and the necessary ability of the cell to change these boundaries.
The challenge in studying the membrane is its complexity. The membrane is a thin sheet of small molecules, i.e., lipids. There are hundreds of types of lipids in the cell. Each lipid changes the properties of the membrane in its vicinity, sometimes making the sheet stiffer, sometimes softer, and sometimes acting to bend the membrane into a ball or tube. Furthermore, the lipids are constantly jostling and tangling, both with each other and with proteins embedded in the membrane. To predict of how membranes are reshaped thus requires not only knowing how lipids affect the properties of the membrane surface, but also the location of specific lipids.
The question as to how molecular scale features influence extensive biological processes must be answered in the language of physical laws. Physics is the language of mechanism at the molecular scale. The challenge is linking physics to the ‘big’ processes that happen in life. Our lab uses detailed physics-driven molecular simulation to ‘build up’ models that can be applied at the much larger level of the cell, which requires retaining important information and eliminating irrelevant details. The software our lab develops is based on the models that we are building. Thus, a broad objective of our research is to create a publicly available software package that can be used either as a stand-alone application for analyzing membrane-reshaping processes or as a library for cellular-scale modeling packages for which the role of the membrane may be unclear or unanticipated.
Another key component of our research is to seek the best possible validation of our models. Few techniques are able to yield molecular information about lipids. Recent breakthroughs that break the diffraction-limit barrier are typically only applicable to static structures much larger than a molecular dye. In contrast, lipids are small and dynamic. Our group is making a sustained effort to validate our simulation findings by applying neutron scattering techniques. This year, our lab initiated new collaborations on the basis of our previous years' work developing methodology for predicting the scattering signal from our simulations.
The projects use the NIH computing resources, including the Biowulf cluster, to run simulations and models. We use molecular dynamics software (such as NAMD and CHARMM) to conduct molecular simulations. In-house software development for public distribution is a key element of the lab's work.
A critical analysis of the crowding mechanism of membrane reshaping
Cellular membranes are reshaped by various mechanisms. A principal mechanism is for a scaffold of curved proteins to bind to the membrane, enforcing their own shape. Proteins and lipids may also directly modify the properties of the surface in which they are embedded, for example, by ‘wedging’ into the surface to which they apply a force to bend. A third mechanism is that of ‘crowding,’ in which a surface, packed with large proteins, curves to lower the density of proteins. In our article [Reference 1], which analyzes the physical forces that are part of crowding, our revision of the theory makes clear that the literature explanation is not valid at the lower (closer to physiological) regime of protein coverage. Instead, we suggest that there is likely an additional interaction of bound proteins with the membrane surface that is independent of crowding.
The biophysics of membrane reshaping in fusion and fission
A key point of our work this year touches on the physics of lipids in membrane fusion and fission, i.e., the process of creating and absorbing vesicles (tiny lipidic spheres) that transport proteins between organelles.
The key physical descriptor of how hard it is to reshape a piece of membrane into a vesicle is the bending modulus, which is an elastic constant similar to what engineers use to describe macroscopic materials. It describes the flexibility of the nanometer-thick sheet of lipids that form a bilayer membrane in the cell. Intuitively, the stiffness of cells' membranes is determined by how thick they are, with saturated fatty tails and cholesterol typically leading to thicker, stiffer membranes. We showed how molecular simulations can identify mechanisms by which lipids can make membranes softer, and thus easier to reshape [Reference 4]. The methodology has the ability to characterize how nanometer-scale patches of lipids can determine the properties of complex biological membranes and thus explain how disorders in lipid synthesis affect the many processes that require membranes to be reshaped.
Our study has guided current projects on how small changes in sterol chemistry lead to significant differences in the outcomes of clathrin-mediated endocytosis. In collaborations, the lab has contributed theory to describe the shape and stability of membranes as they are reshaped by clathrin cages [Reference 2], as well as molecular simulations of amphipathic helices of the immune system protein IFITM3, as part of a project exploring the mechanism of its action blocking viral fusion with our cells [Reference 3].
Publications
- Sapp KC, Sodt AJ. Observed steric crowding at modest coverage requires a particular membrane-binding scheme or a complementary mechanism. Biophy J 2022 121:430–438.
- Guo SK, Sodt AJ, Johnson ME. Large self-assembled clathrin lattices spontaneously disassemble without sufficient adaptor proteins. PLoS Comput Biol 2022 18:e1009969.
- Rahman K, Datta SAK, Beaven AH, Jolley AA, Sodt AJ, Compton AA. Cholesterol binds the amphipathic helix of IFITM3 and regulates antiviral activity. J Mol Biol 2022 434:167759.
- Lessen HJ, Sapp KC, Beaven AH, Ashkar R, Sodt AJ. Molecular mechanisms of spontaneous curvature and softening in complex lipid bilayer mixtures. Biophys J 2022 121:3188–3199.
Collaborators
- Rana Ashkar, PhD, Virginia Tech, Blacksburg, VA
- Alex A. Compton, PhD, HIV Dynamics and Replication Program, Center for Cancer Research, NCI, Frederick, MD
Contact
For more information, email alexander.sodt@nih.gov or visit https://sodtlab.nichd.nih.gov.