Extracellular Matrix Disorders: Molecular Mechanisms and Treatment Targets
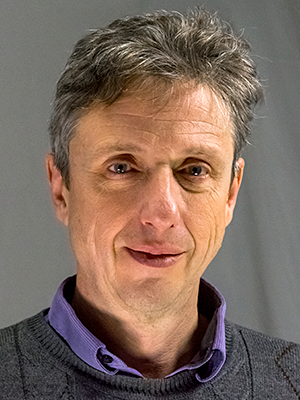
- Sergey Leikin, PhD, Head, Section on Physical Biochemistry
- Elena N. Makareeva, PhD, Staff Scientist
- Edward L. Mertz, PhD, Staff Scientist
- Satoru Otsuru, MD, PhD, Special Volunteer
- Muthulakshmi Sellamani, PhD, Postdoctoral Fellow
- Megan Sousa, BA, Postbaccalaureate Fellow
The extracellular matrix (ECM) is responsible for the structural integrity of tissues and organs as well as for maintaining an optimal environment for cellular function. ECM pathology is involved in a wide variety of disorders, ranging from rare genetic abnormalities of skeletal development (skeletal dysplasias) to such common ailments as osteoporosis, fibrosis, and cancer. Collagens are triple-helical proteins that form the structural scaffolds of the ECM. Their procollagen precursors are assembled and folded from three pro-alpha chains in the endoplasmic reticulum (ER), trafficked through the Golgi apparatus, secreted, and then converted into mature collagen by enzymatic cleavage of propeptides. The most common collagen is type I, which is a heterotrimer of two alpha1(I) chains and one alpha2(I) chain and is by far the most abundant protein in all vertebrates. Type I collagen fibers form the organic scaffold of bone, tendons, ligaments, and the matrix of skin and many other tissues. We focus on translational studies of developmental disorders of the ECM such as osteogenesis imperfecta (OI), Ehlers-Danlos syndrome (EDS), and chondrodysplasias, as well as related ECM pathologies in fibrosis, cancer, and osteoporosis. Our goal is to understand the molecular mechanisms of ECM disorders involving abnormal collagen metabolism, identify treatment targets, and bring this knowledge to clinical research and practice.
Procollagen folding and its role in ECM disorders
Osteoblasts and fibroblasts produce and secrete the massive amounts of type I procollagen needed to build the skeleton and other tissues. Type I procollagen is one of the most difficult proteins to fold. Its massive production presents a unique challenge for protein quality control and trafficking. We discovered that, above 35°C, the conformation of natively folded human procollagen is less favorable than the unfolded one. To fold procollagen at body temperature, cells use specialized ER chaperones to stabilize the native conformation. Outside the cell, the native conformation is stabilized after procollagen is converted to collagen and incorporated into collagen fibers. Unincorporated molecules denature within several hours of secretion and become susceptible to rapid proteolytic degradation. Up to 10–15% of procollagen is misfolded even under normal conditions, necessitating activation of cell stress–response pathways that are responsible for degrading misfolded molecules and which force the cell to always function in a high-stress mode. Our findings indicate that one of the key pathophysiological mechanisms of OI and other hereditary type I collagen–metabolism disorders is cell stress caused by excessive accumulation of misfolded procollagen in the ER.
The most common hereditary cause of procollagen misfolding is a Gly substitution anywhere in the obligatory (Gly-X-Y)n sequence that distinguishes all collagens. Such substitutions in type I collagen are responsible for over 80% of severe OI cases. Similar substitutions in other collagens cause EDS and a variety of other syndromes. Their pathophysiology is one of the key topics of our studies. For instance, our studies on OI patients with over 50 different Gly substitutions revealed several structural regions within the collagen where such mutations might be responsible for distinct OI phenotypes. One such region is the first 85–90 amino acids at the N-terminal end of the triple helix, mutations in which prevent normal N-propeptide cleavage. Incorporation of the uncleaved molecules into collagen fibrils leads to distinct OI/EDS with hyperextensibility and joint laxity.
While we focus mostly on hereditary disorders affecting children, excessive procollagen misfolding may also occur upon changes in the ER associated with environmental factors, inflammation, aging, etc. It is likely to contribute to fibrosis, cancer, age-related osteoporosis, and many other common ailments. Nonetheless, the pathophysiology of misfolded procollagen accumulation in the ER remains poorly understood.
Cell biology of procollagen misfolding
To understand this pathophysiology, we are investigating how misfolded procollagen is recognized by the cell, why it accumulates in the ER, and how this accumulation affects a cell's function. In one approach, we are using live-cell imaging to study the synthesis, folding, trafficking, and degradation of fluorescently tagged procollagen. To facilitate these studies, we created several plasmids enabling transient expression of fluorescent procollagen in mouse and human cells as well as in several cell lines, in which the fluorescent tag was inserted into the gene encoding endogenous pro-alpha2(I) chain. These new tools for live cell imaging of procollagen have already been shared with dozens of laboratories in the USA and around the world.
Imaging of fluorescent procollagen enabled us to demonstrate that normally folded molecules are loaded into Golgi-bound transport vesicles at ER exit sites (ERESs) that are marked by the coat protein complex II (COPII). Contrary to widely held beliefs, however, such vesicles do not have a COPII coat nor do they contain HSP47, a collagen-specific ER chaperone that preferentially binds to natively folded procollagen to assist in its folding and loading into ERESs. Instead, transport-vesicle formation depends on COPI coat assembly and HSP47 release at distal regions of ERESs, potentially explaining unusual skeletal pathologies caused by mutations in HSP47, COPI, and COPII coat proteins. Misfolded procollagen is retained at ERESs, resulting in a COPII–dependent modification of ERES membranes by ubiquitin and autophagic machinery. We discovered that such ERESs are then directly engulfed by lysosomes and degraded, delineating a new ERES micro-autophagy pathway.
Rerouting of ERES–loaded cargo from secretion to micro-autophagy may have wide implications. It is likely to be a general rather than a collagen-specific phenomenon, considering the known COPII coat involvement in both protein secretion and degradation. The hypothesis is currently under investigation in our and several collaborating laboratories. From clinical and translational perspectives, our findings may explain why patients with mutations in different COPII proteins have distinct pathologies in the development of bone, cartilage, and other tissues.
In another approach, we are investigating the cell-stress response to procollagen misfolding caused by a Gly610 to Cys substitution in the triple-helical region of pro-alpha2(I). We helped develop a mouse model of OI with this mutation (G610C mouse), which mimics the pathology found in a large group of patients with the same mutation. Our study of this model demonstrated that misfolding and accumulation of mutant procollagen in the ER of osteoblasts causes ER disruption, resulting in cell stress and malfunction. We are therefore investigating the mechanism of this stress and its role in pathology by altering how the cells adapt to it and by examining cell-stress response pathways activated by the mutation. For instance, we reduced autophagic degradation of ERESs containing misfolded procollagen by osteoblast-specific knockout of ATG5 (autophagy-related factor 5). Increased bone pathology caused by the resulting additional accumulation of misfolded procollagen in the ER confirmed our hypothesis that osteoblast cell stress and malfunction associated with such accumulation play a significant role in OI pathophysiology. More detailed analysis of the ATG5 knockout effects confirmed that ERES microautophagy is the primary pathway of misfolded procollagen degradation in G610C osteoblasts. Furthermore, more recent studies revealed that accumulation of misfolded G610C procollagen in osteoblast ER activates transcription of the integrated stress response genes (e.g., Ddit3, Eif4ebp1, and Nupr1) but not of the canonical ER stress transducers Atf4 and Hspa5, suggesting non-canonical cell stress and identifying its transducers as Atf5 and Hspa9 paralogs of Atf4 and Hspa5. We validated these findings by bulk, single-cell, and spatially resolved RNA sequencing, as well as by in situ RNA hybridization and Western blotting.
By combining the live-cell imaging, genetic, and biochemical analysis, we found that misfolding of procollagen in the ER of G610C osteoblasts activates the mitochondrial arm of the integrated cell stress response (ISR) rather than the canonical unfolded protein response (UPR). Misfolded G610C molecules are not recognized and retained in the ER lumen by quality-control chaperones. Instead, they are retained at ERESs, blocking the exit of all properly folded secretory proteins from the ER into the secretory pathway. The resulting ER overcrowding activates the ISR without UPR as a result of disruption of ER–mitochondria contacts. The ISR is sufficient to stabilize G610C osteoblasts in a less efficient yet functional steady state, in which procollagen synthesis is reduced and degradation is increased. However, the same ISR may not be sufficient to prevent more severe ER disruption in other cells or other mutations, which may then cause misfolding of globular proteins and concomitant secondary UPR. For instance, we found that hypertrophic chondrocytes in the growth plate of the same G610C animals undergo a secondary UPR, which blocks their transition into osteoblasts and thereby limits longitudinal bone growth. Our discovery of mitochondrial involvement not only identified a new response pathway to protein misfolding in the ER but also opened up a new research direction. We are currently investigating how the ER–mitochondria contacts are disrupted and how the resulting mitochondrial dysfunction affects the cells.
New approaches to analysis and treatment of ECM pathology
Our observations suggested that the pathology associated with procollagen misfolding may be partially reversed by improving cell adaptation to misfolded procollagen accumulation in the ER, thereby improving cellular function. Although this would not eliminate the detrimental effects of secreted mutant collagen, pharmacological treatment of cell malfunction is the most realistic short-term strategy for targeting the causes rather than the effect of bone pathology in OI. It is also likely to be a good long-term strategy for the treatment of cell malfunction caused by procollagen misfolding in cases that do not involve pathogenic mutations.
To pursue the strategy, we are examining the effects of enhancing the natural ability of cells to remove and degrade misfolded molecules via autophagy, which is the simplest way to prevent their pathogenic accumulation in the ER. Our studies on autophagy enhancement by a low-protein diet or intermittent fasting in G610C mice revealed improved osteoblast differentiation and function, resulting in better bone quality, but we also observed stunted animal growth. We are thus evaluating other approaches that might provide the same benefits of autophagy enhancement without long-term nutrient deficiency.
In particular, we are testing drugs known to reduce ER disruption by enhancing secretion and autophagy of misfolded proteins (e.g., 4-phenylbutyrate [4PBA]) and drugs (e.g., integrated stress response inhibitor [ISRIB]) known to reduce the impact of accumulating misfolded proteins on overall protein synthesis. We found that 4PBA reduces bone pathology in a zebrafish model of OI and in G610C mice. In such mice, however, low-dose 4PBA treatment improved the function of hypertrophic chondrocytes and their conversion into osteoblasts but not the function of osteoblasts, probably because it alleviated secondary UPR more efficiently than the primary non-canonical cell stress. At the same time, a higher-dosage treatment is challenging because 4PBA is very rapidly metabolized and therefore difficult to deliver in a sustainable fashion to bone cells. We are therefore exploring other drugs, alternative delivery methods, and other approaches.
A key issue in monitoring treatment efficiency in animal models as well as in general diagnostic analysis of bone pathology is the lack of reliable methods with which to characterize the function of bone cells. Traditional histopathology relies on subjective analysis of bone-cell morphology in tissue sections, which is not a reliable indicator of cell function. Over the last several years, we developed a new approach to visualizing and quantifying mRNA expression in individual cells in bone sections. The approach enables objective and reliable cell identification as well as in situ characterization of cell differentiation and function. Based on the interest of bone histomorphometry experts in learning this approach, we hope that it will soon be adapted not only for research but also for clinical practice.
Translational studies of OI and other skeletal dysplasias
Over the last several years, we assisted several clinical research groups in characterizing collagen-metabolism pathology in cells from patients with newly discovered skeletal dysplasias caused by mutations in cartilage-associated protein (CRTAP), prolyl-3-hydrohylase (P3H1), cyclophilin B (CYPB), the collagen-binding molecular chaperone FKBP65, the signaling protein WNT1, the ER–membrane ion channel TRICB, the Golgi-membrane metalloprotease S2P, the transmembrane anterior-posterior transformation protein 1 (TAPT1), or collagen prolyl-4-hydroxylase 1 (P4H1). Our studies suggested that the CRTAP/P3H1/CYPB complex functions as a procollagen chaperone. A deficiency in any of the three proteins delays procollagen folding, although their exact roles remain unclear. More surprisingly, we found no detectable changes in the procollagen folding rate in cultured fibroblasts from patients with FKBP65 mutations. Our data suggested that FKBP65 may affect post-translational modification of procollagen and deposition of collagen matrix by a different mechanism. It remains unclear why some FKBP65 mutations cause severe OI with joint contractures (Bruck's disease), while others cause joint contractures without pronounced OI (Kuskokwim syndrome) or OI without pronounced joint contractures. Our study of TRICB–deficient cells revealed abnormal conformation and reduced thermal stability of type I procollagen, suggesting dysregulation of collagen chaperones in the ER or direct involvement of TRICB in procollagen folding. Our experiments indicated that the pathogenic effects of mutations in the transmembrane protein TAPT1 or in site-2 metalloprotease (S2P) might not be directly related to disruptions in synthesis, folding, or trafficking of procollagen chains. As expected, we found that patient cells with mutant P4H1 secreted abnormal procollagen, which had significantly reduced thermal stability owing to under-hydroxylation of proline residues by P4H1. Surprisingly, however, we found no abnormalities in the procollagen folding or secretion rates, no evidence of misfolded procollagen accumulation in the cell, and no evidence of altered ER chaperone composition.
We also conducted translational studies of OI caused by missense mutations in type I collagen that are not substitutions of obligatory Gly residues. For instance, we found that substitutions of Y-position arginine (Y-Arg) residues in the Gly-X-Y triplets within the collagen triple helix cause procollagen misfolding and accumulation in the ER to almost the same extent as Gly substitutions, likely because Y-Arg enhances collagen triple-helix stability and promotes triple-helix folding through binding of HSP47.
Presently, we are focusing on understanding the causes of lung pathology in patients and mouse models of OI. Our micro–CT, histological, and transcriptomic studies revealed lung tissue pathology in G610C mice and two other mouse models of OI that could not be related to skeletal deformities. Given that cardio-pulmonary complications are the main cause of death in OI, we are investigating how disruptions of collagen metabolism in lung tissue are causing the pathology. Our preliminary experiments have already uncovered abnormal cell–ECM and cell-cell interactions affecting alveologenesis, mechanical strength of the tissue, and differentiation of lung epithelial cells. Beyond a better fundamental understanding of cell–ECM and cell-cell interactions in lungs, we hope that these studies will identify potential therapeutic targets.
Extracellular matrix pathology in tumors and fibrosis
Another aspect of our collagen metabolism pathology studies has been to characterize the pathology in fibromas and tumors, e.g., abnormal collagen composition of uterine fibroids and the potential role of type I collagen homotrimers in cancer. The normal isoform of type I collagen is a heterotrimer of two alpha1(I) chains and one alpha2(I) chain. Homotrimers of three alpha1(I) chains are produced in some fetal tissues, carcinomas, fibrotic tissues, as well as in rare forms of OI and EDS associated with alpha2(I) chain deficiency. We found the homotrimers to be at least 5–10 times more resistant to cleavage by all mammalian collagenases than the heterotrimers, and we determined the molecular mechanism of this resistance. Our studies suggested that cancer cells might utilize this collagen isoform to build collagenase-resistant tracks, thus supporting invasion through stroma of lower resistance.
We also investigated bone pathology and tumors caused by defects in cAMP signaling, e.g., those associated with mutation in protein kinase A (PKA), which is a key enzyme in the cAMP signaling pathway. Initially, we studied synthesis of type I collagen homotrimers. However, over the last 3–5 years, our focus shifted to abnormal differentiation of osteoblastic cells and deposition of bone. We found that knockouts of various PKA subunits cause not only abnormal organization and mineralization of bone matrix but also novel bone structures that had not been previously reported. For instance, we observed free-standing cylindrical bone spicules with an osteon-like organization of lamellae and osteocytes but an inverted mineralization pattern, a highly mineralized central core, and diminishing mineralization away from the central core. We assisted clinical researchers in characterizing abnormal osteoblast maturation, the role of an abnormal inflammatory response, and effects of anti-inflammatory drug treatments in such animals. Improved understanding of bone tumors caused by PKA deficiencies may not only clarify the role of cAMP signaling but may also suggest new approaches to therapeutic manipulation of bone formation in skeletal dysplasias.
Multimodal imaging and mapping of tissues
Given that tissue analysis is crucial for understanding and treating collagen metabolism disorders, we are developing methods for characterizing cellular function in relation to ECM composition and structure. The methodology builds on our advances in high-definition infrared and Raman microspectroscopy, mRNA–based histology and histomorphometry, and combining different imaging and spectroscopic modalities for tissue sections.
In particular, we developed high-definition (HD) infrared imaging and Raman micro-spectroscopic methods, improving spectral reproducibility by up to two orders of magnitude, based on thermomechanical stabilization of the light path through tissue. The technology enabled us to uncover the causes of progressive cartilage degradation in a mouse model of diastrophic dysplasia caused by mutations in the SLC26A2 sulfate transporter gene. It was essential for the analysis of abnormal collagen matrix deposition by CRTAP– and FKBP65–deficient cells. We used it in studies of bone structure and mineralization in the mouse models of the OI and PKA deficiencies described above and for establishing new approaches to characterizing bone formation in vitro. Beyond nondestructive characterization of ECM composition and structure in many of our studies, the technology proved to be useful for researchers from other NICHD and NIH laboratories. For instance, we assisted NIBIB scientists in characterizing a functionalized carbon-nanotube approach to the delivery of anticancer agents into cells that overexpress hyaluronate receptors.
We are presently working on integrating the HD infrared/Raman and histological ECM imaging modalities with cellular function imaging based on in situ mRNA sequencing and fluorescent in situ mRNA hybridization. We have already combined all these modalities for proving formation of bona fide lamellar bone in osteoblast cultures and developing approaches to distinguishing this bone from other mineralized cell–ECM structures, which are generally more prevalent in vitro. We are now optimizing such structural and functional imaging for integrated characterization of the same tissue section, which would address the question of how the ECM structure and composition affect the function of adjacent cells and cell-cell interactions.
Publications
- Omari S, Makareeva E, Leikin S. Procollagen trafficking and its implications in osteogenesis imperfecta. In: The Collagen Superfamily and Collagenopathies, Ruggiero F, ed. 2021 Chapter 2:23–53.
- Gorrell L, Omari S, Makareeva E, Leikin S. Noncanonical ER–Golgi trafficking and autophagy of endogenous procollagen in osteoblasts. Cell Mol Life Sci 2021 78:8283.
- Scheiber AL, Wilkinson KJ, Suzuki A, Enomoto-Iwamoto M, Kaito T, Cheah KS, Iwamoto M, Leikin S, Otsuru S. 4PBA reduces growth deficiency in osteogenesis imperfecta by enhancing transition of hypertrophic chondrocytes to osteoblasts. JCI Insight 2022 7:e149636.
- Gorrell L, Makareeva E, Omari S, Otsuru S, Leikin S. Mitochondria, and ISR regulation by mt-HSP70 and ATF5 upon procollagen misfolding in osteoblasts. Adv Sci (Weinh) 2022 9:2201273.
- Mertz EL, Makareeva E, Mirigian LS, Leikin S. Bone formation in 2D culture of primary cells. JBMR Plus 2022; https://doi.org/10.1002/jbm4.10701.
Collaborators
- Peter Basser, PhD, Section on Quantitative Imaging and Tissue Sciences, NICHD, Bethesda, MD
- Peter H. Byers, MD, University of Washington, Seattle, WA
- Antonella Forlino, PhD, Università degli Studi di Pavia, Pavia, Italy
- Ken Kozloff, PhD, University of Michigan, Ann Arbor, MI
- Jennifer A. Lippincott-Schwartz, PhD, Howard Hughes Medical Institute, Janelia Farm Research Campus, Ashburn, VA, and Adjunct Investigator, NICHD, Bethesda, MD
- Fransiska Malfait, MD, PhD, Center for Medical Genetics, Ghent University, Ghent, Belgium
- Joan C. Marini, MD, PhD, Section on Heritable Disorders of Bone and Extracellular Matrix, NICHD, Bethesda, MD
- Roy Morello, PhD, University of Arkansas Medical School, Little Rock, AR
- Cathleen Raggio, MD, Hospital for Special Surgery, New York, NY
- Pamela G. Robey, PhD, Craniofacial and Skeletal Diseases Branch, NIDCR, Bethesda, MD
- Robert Sandhaus, MD, PhD, National Jewish Health, Denver, CO
- Sofie Symoens, PhD, Center for Medical Genetics, Ghent, Ghent University, Belgium
Contact
For more information, email leikins@mail.nih.gov or visit https://physbiochem.nichd.nih.gov.