Molecular Nature and Functional Role of Dendritic Voltage-Gated Ion Channels
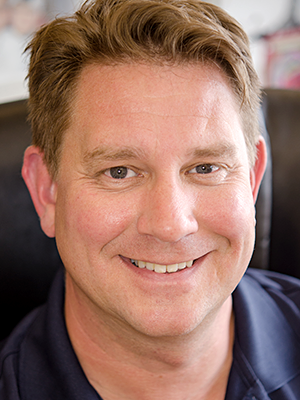
- Dax Hoffman, PhD, Head, Molecular Neurophysiology and Biophysics Section
- Jiahua Hu, PhD, Staff Scientist
- Lin Lin, PhD, Microbiologist
- Ying Liu, MD, Biologist
- Cole Malloy, PhD, Postdoctoral Fellow
- Jon Murphy, PhD, Postdoctoral Research Associate Program (PRAT) Fellow
- Adriano Bellotti, BS, Gates Cambridge Scholar
- Joseph Krzeski, BS, Postbaccalaureate Fellow
The central nervous system (CNS) underlies all our experiences, actions, emotions, knowledge, and memories. With billions of neurons each firing hundreds of times per second, the complexity of the brain is stunning. To pare down the task of understanding something so complex, our research approach calls for studying the workings of a single central neuron: the pyramidal neuron from the CA1 region of the hippocampus. The hippocampus is essential for long-term memory in humans and is among the first brain regions affected by epilepsy and Alzheimer’s disease. To understand how the hippocampus stores and processes information, we focus on one of its principal cell types, the CA1 pyramidal neuron. Each pyramidal neuron in the CA1 region of the hippocampus receives tens of thousands of inputs onto its dendrites, and it is commonly thought that information is stored by altering the strength of individual synapses (synaptic plasticity). Recent evidence suggests that the regulation of synaptic surface expression of glutamate receptors can, in part, determine synaptic strength. However, the dendrites contain an abundance of ion channels that are involved in receiving, transforming, and relaying information in the dendrites, adding an additional layer of complexity to neuronal information processing.
We found that the A-type potassium channel subunit Kv4.2 is highly expressed in the dendritic regions of CA1 neurons in the hippocampus and, as one of the primary regulators of dendritic excitability, plays a pivotal role in information processing. Kv4.2 is targeted for modulation during the types of plasticity thought to underlie learning and memory. Moreover, we found that the functional expression level of Kv4.2 regulates the subtype expression of NMDA–type glutamate receptors, the predominant molecular devices controlling synaptic plasticity and memory. We are currently following up on these findings with more detailed investigations into the mechanisms of activity-dependent Kv4.2 regulation. In addition, we have begun to investigate the role of dendritic voltage-gated potassium and calcium channels in neuronal development and developmental disorders.
Role of voltage-gated ion channels in synaptic development and disease
Regulation of potassium channel trafficking and function
Kv4.2 channels, the major contributors to somatodendritic A-type potassium channels, are key determinants of dendritic excitability and integration, spike timing–dependent plasticity, and long-term potentiation. Downregulation of Kv4.2 channel expression occurs following hippocampal seizures and in epilepsy, suggesting that A-type currents may be targets for novel therapeutics. To identify Kv4.2 binding proteins, Jiahua Hu employed a tandem affinity purification approach (TAP) to isolate the Kv4.2 protein complex from hippocampal neurons. Mass-spectrometry (MS) analysis identified known proteins such as KChIP family members and DPP6/10. The TAP–MS assay also identified an isomerase as a binding partner of Kv4.2. The binding was confirmed by brain co-immunoprecipitation, co-expression in HEK293T cells, and peptide pull-down in vitro. The isomerase binds to a specific Kv4.2 site, and the association is regulated by neuronal activity and seizure.
To determine whether and how the isomerase regulates the trafficking of Kv4.2, former Postbaccalaureate Fellow Travis Tabor generated bungarotoxin binding site–tagged Kv4.2 at the second extracellular loop for visualizing Kv4.2 in live neurons while in our lab. In biochemical and electrophysiological assays, the bungarotoxin binding site–tagged Kv4.2 showed similar channel properties to those of wild-type (WT) Kv4.2. The isomerizing activity may also regulate Kv4.2 binding to its auxiliary subunits. These data suggest that the isomerase plays a role in regulating Kv4.2 function.
To further study the physiological function of the isomerase and the Kv4.2 channel, we used Crispr-Cas9 techniques to generate a knockin (KI) mouse in which the isomerase binding site is specifically abolished. The mice are viable, appear normal, and showed normal initial learning and memory in Morris Water Maze but better 'reversal' learning in Morris Water Maze than did WT mice. In the operant reversal lever press, the KI mice displayed improved reversal learning. The data strongly support the idea that activity-dependent regulation of Kv4.2 plays an important role in cognitive flexibility. Cognitive flexibility is the ability to appropriately adjust one’s behavior to a changing environment and is impaired in various neurodevelopmental disorders such as the autism spectrum disorder (ASD).
In light of these findings, Cole Malloy used whole-cell patch clamp electrophysiology in acute hippocampal slices to investigate how isomerization of Kv4.2 impacts neuronal function. He used current-clamp recordings to detect alterations in action-potential firing properties in the KI mice. In order to gain further insights into the molecular cascade impacting Kv4.2 function, pharmacological manipulation of isomerase and kinase activity are under way to address the dependence of phosphorylation and/or conformation change induced by the isomerase. Given the behavioral results showing altered cognitive flexibility, we plan experiments investigating synaptic function and plasticity in the KI mice.
In an effort to study Kv4.2 trafficking in the context of ion channel homeostasis during perturbations to neuron intrinsic excitability, we discovered quantitative and qualitative differences in microtubule-based transport in axons versus dendrites. Adriano Bellotti characterized the differences by recording time series of over 500 neurites, and we validated an unexpected result using mathematical models of cargo transport. We developed a deterministic model that corroborates differences in cargo frequency and a stochastic model that validates differences in puncta speed, superdiffusivity, and frequency in axons vs dendrites. In a secondary project, we are studying deterministic models of nonlinear cargo transport with feedback control and with branching. We are now embarking on such simulations with realistic pyramidal cell morphologies.
Travis Tabor developed a novel tool for labeling only the cell-surface fraction of Kv4.2 channels. The tool will be useful for studying surface expression levels and surface trafficking of Kv4.2 channels.
Ca2+ regulation of potassium channel function
In addition to pore-forming Kv4 subunits, native hippocampal A-type currents require non-conducting modulatory auxiliary subunits known as K-channel–interacting proteins (KChIPs) and dipeptidyl peptidase–like proteins (DPLPs). Both KChIPs and DPLPs work in concert to enhance Kv4 function. Interestingly, in recent unpublished work, we identified a mechanism by which Kv4.2 current density is regulated by Ca2+ via R-type voltage-gated Ca2+ channels (Cav2.3). Ca2+ regulation of Kv4.2 channels occurs despite an apparent lack of the structural determinants of the canonical Ca2+-activated K+ channels. We hypothesized that KChIP auxiliary proteins imbue Kv4 channels with Ca2+ sensitivity, as they contain four calcium-binding EF–hand domains (helix-loop-helix structural domains), two of which bind Ca2+.
To assess this possibility, Jon Murphy expressed KChIP2c, a short KChIP isoform, with Kv4.2 in HEK293T cells and performed whole-cell patch-clamp recordings in either nominal or 10 micromolar Ca2+. Under low Ca2+ conditions, coexpression of KChIP2c enhances Kv4.2 function in several ways: KChIP2c increases K+ current density, shifts the voltage dependence of inactivation to more hyperpolarized potentials, and accelerates recovery from inactivation. In the presence of 10 micromolar Ca2+, he measured a 1.5 fold increase in peak Kv4.2 current density while the other parameters of Kv4 function remained unchanged. Intriguingly, whereas the boosting effect of Ca2+ is conserved among the Kv4 family, including Kv4.1-4.3, it was only observed for short splice isoforms of KChIP2, KChIP2b/c, and the predominant KChIP3 isoform, KChIP3a. KChIP mRNAs contain many start sites, which generate considerable N-terminal variation and functional diversity in shaping A-type currents. While the KChIP core is cytoplasmic by nature, the variable N-termini regulate subcellular localization by encoding variable membrane-interaction motifs. Comparisons of the variable N-terminal domains of KChIP2 isoforms suggested that a conserved polybasic domain limits the Ca2+ sensitivity of longer KChIP2 isoforms. Ongoing studies are aimed at determining whether this previously unidentified polybasic domain regulates the plasma membrane trafficking and Ca2+ sensitivity of Kv4–KChIP2 complexes.
Proteomic and subcellular localization studies suggest that Cav2.3–containing voltage-gated calcium channels are a potential calcium source for a modulatory effect on Kv4.2–mediated A-type K currents (IA) in CA1 hippocampal neurons. While in our lab, former Visiting Fellow Jakob Gutzmann compared WT with Cav2.3 knock-out (KO) neurons and observed a significant reduction in somatic IA. Cav2.3 KO neurons showed an elevated frequency of action potential firing after somatic injection of positive current. Further investigation revealed that individual action potentials showed profound changes in waveform: longer time-to-peak, and more significantly, a broader full width at half-maximum. Additionally, the fast after-hyperpolarization following an action potential was shifted towards positive potentials in cells from the KO animals. Ultimately, he could track these changes pharmacologically to a functional lack of large-conductance potassium (BK) channel activity. This is the first time that Cav2.3 has been linked to BK channel activity, which so far has been thought to be regulated by other calcium channels. To support this novel finding, Lin Lin also performed co-immunoprecipitation (co-IP) experiments from acutely isolated adult mouse hippocampal tissue, and could successfully co-IP BK and Cav2.3, which hints at a physical interaction between the two channels, in addition to the functional interaction described above. A consequence of the altered action potential waveform in Cav2.3 KO neurons is an increase in short-term plasticity between CA1 and the informationally downstream-lying subiculum pyramidal neurons.
We found that signaling through metabotropic glutamate receptors supports activity-dependent translation of the voltage-gated calcium channel Cav2.3. The regulation is dependent on the Fragile X mental retardation protein (FMRP) and is therefore lost in FMRP KO mice. The KO mice exhibit exaggerated levels of Cav2.3, which likely contributes to the dendritic hyperexcitability found by other groups.
DPP6 plays a role in brain development, function, and behavior.
Well known as an auxiliary subunit of Kv4.2, DPP6 has been associated with numerous developmental and intellectual disorders and neuropsychiatric pathologies, especially ASD. We previously reported a novel role for DPP6 in regulating dendritic filopodia formation and stability, affecting synaptic development and function. Recently, we reported that DPP6–knockout mice are impaired in hippocampus-dependent learning and memory. Results from the Morris water maze, T-maze, object’s spatial location, novel object recognition, and fear conditioning tasks showed that DPP6–KO mice exhibit slower learning and reduced memory performance. More recently, we observed that DPP6 KO mice have significantly smaller brains than control mice. To determine which regions are affected, we performed in vivo MRI to scan the live mouse brain. Our results showed that DPP6–KO mice have significantly reduced volume specifically in the hippocampus and cerebellum. Our findings indicate that DPP6 loss drives microcephaly and learning and memory impairment in DPP6–KO mice.
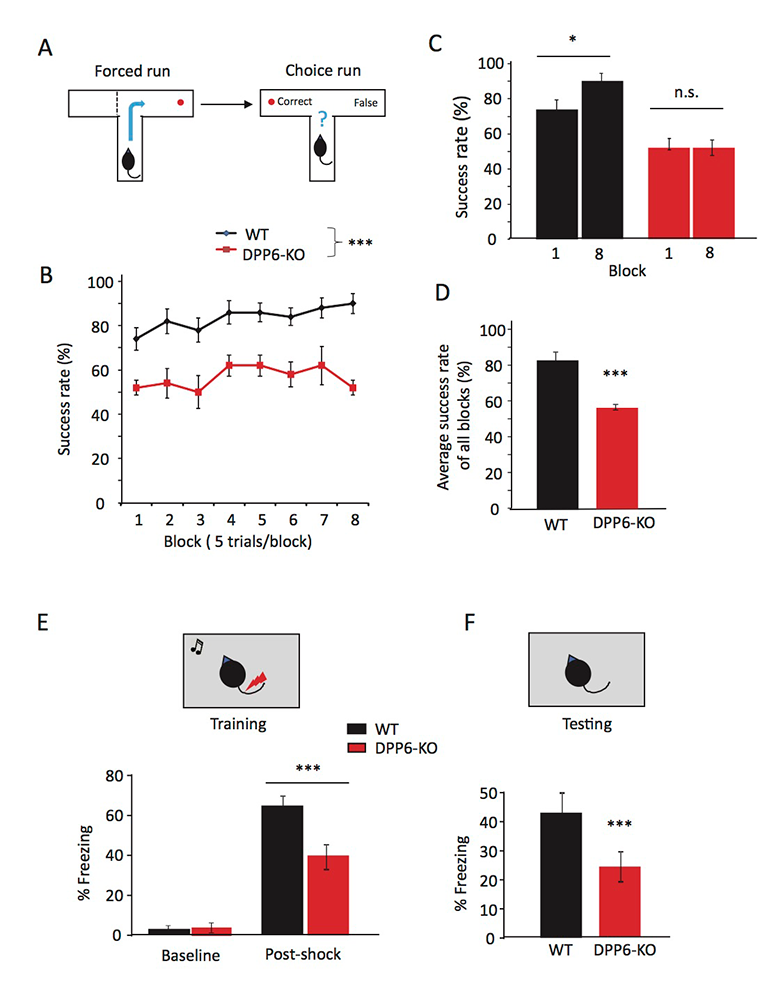
Click image to view.
Figure 1. DPP6-KO mice show impaired spatial working memory in the T-maze and contextual fear conditioning task.
A. Schematic showing the experimental design in forced run and choice run of the T-maze task.
B. Percentage of correct choices for mice during the learning phase.
C. Percentage of correct choices comparing Day 1 with Day 8.
D. Percentage of correct choices for average scores over 8 days (n = 10 each group; p < 0.001), compared with Student’s t-test.
E–F. Contextual fear conditioning task, at 24 hr after training mice, shows a significant reduction in time exhibiting freezing behavior when placed back in the test chamber (n = 10 for each group; p < 0.001).
Additional Funding
- Postdoctoral Research Associate Program (PRAT) Fellowship (Jon Murphy)
- Gates Cambridge Scholarship (Adriano Bellotti)
Publications
- Gray EE, Murphy JG, Liu Y, Trang I, Tabor GT, Lin L, Hoffman DA. Disruption of GpI mGluR-dependent Cav2.3 translation in a mouse model of fragile X syndrome. J Neurosci 2019;39(38):7453-7464.
- Murphy JG, Hoffman DA. A polybasic motif in alternatively spliced KChIP2 isoforms prevents Ca2+ regulation of Kv4 channels. J Biol Chem 2019;294(10):3683-3695.
- Gutzmann JJ, Lin L, Hoffman DA. Functional coupling of Cav2.3 and BK potassium channels regulates action potential repolarization and short-term plasticity in the mouse hippocampus. Front Cell Neurosci 2019;13:27.
- Tabor GT, Park JM, Murphy JG, Hu JH, Hoffman DA. A novel bungarotoxin binding site-tagged construct reveals MAPK-dependent Kv4.2 trafficking. Mol Cell Neurosci 2019;98:121-130.
- Lin L, Murphy JG, Karlsson RM, Petralia RS, Gutzmann JJ, Abebe D, Wang YX, Cameron HA, Hoffman DA. DPP6 loss impacts hippocampal synaptic development and induces behavioral impairments in recognition, learning and memory. Front Cell Neurosci 2018;12:84.
Collaborators
- Avindra Nath, MD, Translational Neuroscience Center, NINDS, Bethesda, MD
- Constantine A. Stratakis, MD, D(med)Sci, Section on Endocrinology and Genetics, NICHD, Bethesda, MD
- Paul Worley, MD, The Johns Hopkins University, Baltimore, MD
Contact
For more information, email hoffmand@mail.nih.gov or visit http://hoffmanlab.nichd.nih.gov.