Studies on DNA Replication, Repair, and Mutagenesis in Eukaryotic and Prokaryotic Cells
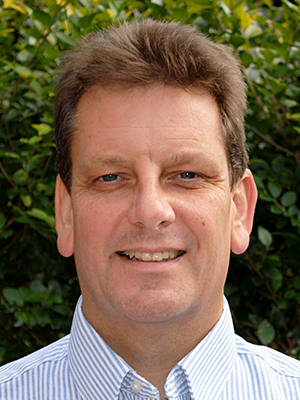
- Roger Woodgate, PhD, Chief, Section on DNA Replication, Repair, and Mutagenesis
- Ekaterina Chumakov, PhD, Staff Scientist
- Alexandra Vaisman, PhD, Interdisciplinary Scientist
- John McDonald, PhD, Biologist
- Mary McLenigan, BS, Chemist
- Nicholas Ashton, PhD, Postdoctoral Visiting Fellow
- In Soo Jung, PhD, Postdoctoral Visiting Fellow
- Katherine Mnuskin, BS, Postbaccalaureate Intramural Research Training Award Fellow
- Kristiana Moreno, BS, Postbaccalaureate Intramural Research Training Award Fellow
- Dominic R. Quiros, BS, Postbaccalaureate Intramural Research Training Award Fellow
- Nicole Wilkinson, BS, Postbaccalaureate Intramural Research Training Award Fellow
- Maya Kaplan, Stay-in-School Intramural Research Training Award Fellow
Under optimal conditions, the fidelity of DNA replication is extremely high. Indeed, it is estimated that, on average, only one error occurs for every 10 billion bases replicated. However, given that living organisms are continually subjected to a variety of endogenous and exogenous DNA–damaging agents, optimal conditions rarely prevail in vivo. While all organisms have evolved elaborate repair pathways to deal with such damage, the pathways rarely operate with 100% efficiency. Thus, persisting DNA lesions are replicated, but with much lower fidelity than in undamaged DNA. Our aim is to understand the molecular mechanisms by which mutations are introduced into damaged DNA. The process, commonly referred to as translesion DNA synthesis (TLS), is facilitated by one or more members of the Y-family of DNA polymerases, which are conserved from bacteria to humans. Based on phylogenetic relationships, Y-family polymerases may be broadly classified into five subfamilies: DinB–like (polIV/pol kappa–like) proteins are ubiquitous and found in all domains of life; in contrast, the Rev1–like, Rad30A (pol eta)–like, and Rad30B (pol iota)–like polymerases are found only in eukaryotes; and the UmuC (polV)–like polymerases only in prokaryotes. We continue to investigate TLS in all three domains of life: bacteria, archaea, and eukaryotes.
Prokaryotic mutagenesis
As part of an international scientific collaboration with Antoine van Oijen and Michael Cox, we investigated the subcellular localization of the E.coli RecA protein, which orchestrates the cellular response to DNA damage via its multiple roles in the bacterial SOS response. The lack of tools to provide unambiguous access to the various RecA states within the cell has previously prevented an understanding of the spatial and temporal changes in RecA structure/function that underlie control of the DNA damage response. To circumvent these problems, Antoine van Oijen’s group developed a monomeric C-terminal fragment of the λ repressor as a novel fluorescent probe that specifically interacts with RecA filaments on single-stranded DNA (RecA*). Single-molecule imaging techniques in live cells revealed that RecA is largely sequestered in storage structures during normal metabolism. Upon DNA damage, the storage structures dissolve, and the cytosolic pool of RecA rapidly nucleates to form early SOS–signaling complexes, maturing into DNA–bound RecA bundles at later time points. Both before and after SOS induction, RecA* largely appears at locations distal from replisomes. Interestingly, upon completion of DNA repair, RecA storage structures reform [Reference 1].
In a collaboration with Myron Goodman and Michael Cox, we investigated how E.coli DNA polymerase V (pol V) is regulated by a conformational change upon interacting with ATP and the RecA protein). Mutagenic translesion DNA polymerase V (UmuD'2C) is induced as part of the DNA damage–induced SOS response in Escherichia coli, and is subjected to several levels of regulation. The UmuC subunit is sequestered on the cell membrane (spatial regulation) and enters the cytosol after forming a UmuD'2C complex, about 45 min after SOS induction (temporal regulation). However, DNA binding and synthesis cannot occur until pol V interacts with a RecA nucleoprotein filament (RecA*) and ATP to form the mutasome complex pol V Mut (UmuD'2C-RecA-ATP). The location of RecA relative to UmuC determines whether pol V Mut is catalytically on or off (conformational regulation). In our collaborative study, we reported three interrelated experiments to address the biochemical basis of conformational regulation. We first investigated dynamic deactivation during DNA synthesis and static deactivation in the absence of DNA synthesis. Single-molecule (sm) TIRF-FRET microscopy was then used to explore several aspects of pol V Mut dynamics. Binding of ATP/ATPγS triggers a conformational switch that reorients RecA relative to UmuC to activate pol V Mut. The process is required for polymerase–DNA binding and synthesis. Both dynamic and static deactivation processes are governed by temperature and time, in which on to off switching is "rapid" at 37°C (about 1 to 1.5 h), "slow" at 30°C (about 3 to 4 h) and does not require ATP hydrolysis. We found that Pol V Mut retains RecA in both activated and deactivated states but binding to a primer-template (p/t) DNA occurred only when Pol V Mut is activated [Reference 2].
In another study related to polV, we generated a “so-called” steric gate mutant of pol VICE391 found on the integrating conjugative element ICE391 (formerly known as IncJ R391), which incorporates high levels of ribonucleotides into the E. coli genome to investigate the molecular mechanisms of ribonucleotide excision repair (RER). The study was part of an international scientific collaboration with Andrew Robinson, Myron Goodman, and Iwona Fijalkowska. As noted above, pol VICE391 (RumA'2B) is a low-fidelity polymerase that promotes considerably higher levels of spontaneous “SOS–induced” mutagenesis than the related E. coli pol V (UmuD'2C). The molecular basis for the enhanced mutagenesis was previously unknown. Using single-molecule fluorescence microscopy to visualize pol V enzymes, we discovered that the elevated levels of mutagenesis are likely attributable, in part, to prolonged binding of RumB to genomic DNA, leading to higher levels of DNA synthesis than with UmuC. We then generated a steric-gate pol VICE391 variant (pol VICE391_Y13A) that readily misincorporated ribonucleotides into the E. coli genome and used the enzyme to investigate the molecular mechanisms of RER under conditions of elevated ribonucleotide-induced stress. To do so, we compared the extent of spontaneous mutagenesis promoted by E. coli pol V and pol VICE391 with that of their respective steric gate variants. Levels of mutagenesis promoted by the steric gate variants that were lower than that of the wild-type enzyme were indicative of active RER, which removes misincorporated ribonucleotides but also misincorporated deoxyribonucleotides from the genome. Using such an approach, we confirmed that RNase HII plays a pivotal role in RER. In the absence of RNase HII, nucleotide excision repair (NER) proteins help remove misincorporated ribonucleotides. However, significant RER occurred in the absence of RNase HII and NER. Most of the RNase HII and NER–independent RER occurred on the lagging strand during genome duplication. We suggest that this is most likely attributable to efficient RNase HI–dependent RER, which recognizes the polyribonucleotide tracts generated by pol VICE391_Y13A. These activities are critical for the maintenance of genomic integrity when RNase HII is overwhelmed, or inactivated, as demonstrated by the observation that delta rnhB or delta rnhB delta uvrA strains expressing pol VICE391_Y13A exhibited genome and plasmid instability in the absence of RNase HI [Reference 3].
Eukaryotic mutagenesis
We extended our RER studies to Saccharomyces cerevisiae in a collaboration with Anders Clausen. To do so, we utilized a steric-gate mutant of DNA polymerase η (pol η) that we had previously generated in our lab in 2015. Although best known for its ability to bypass UV-induced thymine-thymine (T-T) dimers and other bulky DNA lesions, pol η also has other cellular roles. In our study, we presented evidence that pol η competes genome-wide with DNA polymerases α and δ for the synthesis of the lagging strand, where it also shows a preference for T-T in the DNA template. Moreover, we found that the C-terminus of pol η, which contains a proliferating cell nuclear antigen (PCNA)–Interacting Protein motif is required for pol η to function in lagging-strand synthesis. We also presented evidence that a pol η–dependent signature is also found to be lagging-strand–specific in patients with skin cancer. Taken together, the findings provided insight into the physiological role of DNA synthesis by pol η and have implications for our understanding of how our genome is replicated to avoid mutagenesis, genome instability, and cancer [Reference 4].
In another international scientific collaboration with Justyna McIntyre, we investigated the regulation of human DNA polymerase iota (Polι), which was discovered by scientists in our lab twenty-one years ago. DNA polymerase iota (Polι) belongs to the Y-family of DNA polymerases that are involved in DNA–damage tolerance through their role in translesion DNA synthesis. Like all other Y-family polymerases, Polι interacts with PCNA, Rev1, ubiquitin and ubiquitinated PCNA and is also ubiquitinated itself. In our collaborative study, we reported that Polι also interacts with the p300 acetyltransferase and is acetylated. The primary acetylation site is K550, located in the Rev1–interacting region. However, K550 amino acid substitutions have no effect on Polι's ability to interact with Rev1. Interestingly, we found that acetylation of Polι significantly and specifically increased in response to SN2 alkylating agents and, to a lower extent, to SN1 alkylating and oxidative agents. Given that we did not observe acetylation of Polι's closest paralog, DNA polymerase eta (Polη), with which Polι shares many functional similarities, we believe that acetylation of Polι might exclusively regulate as yet to be determined and separate function(s) of Polι [Reference 5].
Additional Funding
- Collaborative extramural U01 grant (U01HD085531-01) with Prof. Digby Warner, University of Cape Town, South Africa: Replisome dynamics in M. tuberculosis: linking persistence to genetic resistance
Publications
- Ghodke H, Paudel BP, Lewis JS, Jergic S, Gopal K, Romero ZJ, Wood EA, Woodgate R, Cox MM, van Oijen AM. Spatial and temporal organization of RecA in the Escherichia coli DNA-damage response. eLife 2019;8:e4276.
- Jaszczur MM, Vo DD, Stanciauskas R, Bertram JG, Sikand A, Cox MM, Woodgate R, Mak CH, Pinaud F, Goodman MF. Conformational regulation of Escherichia coli DNA polymerase V by RecA and ATP. PLoS Genet 2019;15(2):e1007956.
- Walsh E, Henrikus SS, Vaisman A, Makiela-Dzbenska K, Armstrong TJ, Lazowski K, McDonald JP, Goodman MF, van Oijen AM, Jonczyk P, Fijalkowska IJ, Robinson A, Woodgate R. Role of RNase H enzymes in maintaining genome stability in Escherichia coli expressing a steric-gate mutant of pol VICE391. DNA Repair (Amst) 2019;84:102685.
- Kreisel K, Engqvist MKM, Kalm J, Thompson LJ, Boström M, Navarrete C, McDonald JP, Larsson E, Woodgate R, Clausen AR. DNA polymerase eta contributes to genome-wide lagging strand synthesis. Nucleic Acids Res 2019;47(5):2425-2435.
- McIntyre J, Sobolewska A, Fedorowicz M, McLenigan MP, Macias M, Woodgate R, Sledziewska-Gojska E. DNA polymerase iota is acetylated in response to SN2 alkylating agents. Sci Rep 2019;9(1):4789.
Collaborators
- Anders R. Clausen, PhD, Göteborgs Universitet, Göteborg, Sweden
- Michael Cox, PhD, University of Wisconsin, Madison, WI
- Iwona Fijlakowska, PhD, Polish Academy of Sciences, Warsaw, Poland
- Myron F. Goodman, PhD, University of Southern California, Los Angeles, CA
- Karolina Makiela-Dzbenska, PhD, Polish Academy of Sciences, Warsaw, Poland
- Justyna McIntyre, PhD, Polish Academy of Sciences, Warsaw, Poland
- Andrew Robinson, PhD, University of Wollongong, Wollongong, Australia
- Ewa Sledziewska-Gojska, PhD, Polish Academy of Sciences, Warsaw, Poland
- Antoine Van Oijen, PhD, University of Wollongong, Wollongong, Australia
- Digby Warner, PhD, University of Cape Town, Cape Town, South Africa
- Wei Yang, PhD, Laboratory of Molecular Biology, NIDDK, Bethesda, MD
Contact
For more information, email woodgate@.nih.gov or visit http://sdrrm.nichd.nih.gov.