Modeling the Biophysics of the Membrane
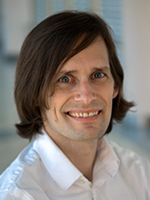
- Alexander J. Sodt, PhD, Head, Unit on Membrane Chemical Physics
- Andrew Beaven, PhD, Postdoctoral Fellow
- Kayla Sapp, PhD, Postdoctoral Fellow
- Victor Chernomordik, PhD, Volunteer
- Mitchell Dorrell, BS, Predoctoral Fellow
- Ruchita Kothari, Summer Intern
- Gudrun Thorkelsdottir, Summer Student
- Igor Semyonov, BS, Summer Student
The integrity of lipid membranes is essential for life. They provide spatial separation of the chemical contents of the cell and thus make possible the electrical and chemical potential differences that are used to transmit signals and perform work. However, the membrane must be broken frequently to form, for example, new membrane structures in the cell. The simplest structure is a vesicle to transport cargo. Such vesicles are constantly cycled between organelles and the outer plasma membrane. Thus, there is a careful balance between boundary-establishing membrane fidelity and the necessary ability of the cell to change these boundaries.
The challenge in studying the membrane is its complexity. The membrane is a thin sheet of small molecules, i.e., lipids. There are hundreds of types of lipids in the cell. Each lipid changes the properties of the membrane in its vicinity, sometimes making the sheet stiffer, sometimes softer, and sometimes acting to bend the membrane into a ball or tube. Furthermore, the lipids are constantly jostling and tangling with both each other and with proteins embedded in the membrane. To predict of how membranes are reshaped thus requires not only knowing how lipids affect the properties of the membrane surface, but also the location of specific lipids.
The question as to how molecular scale features influence extensive biological processes must be answered in the language of physical laws. Physics is the language of mechanism at the molecular scale. The challenge is linking these physics to "big" processes that happen in life. Our Unit uses detailed physics-driven molecular simulation to "build up" models that can be applied at the much larger level of the cell, which requires retaining important information and eliminating irrelevant details. The software our lab develops is based on the models that we are building. Thus, a broad objective of our research is to create a publicly available software package that can be used either as a stand-alone application for analyzing membrane-reshaping processes or as a library for cellular-scale modeling packages for which the role of the membrane may be unclear or unanticipated.
Another key component of our research is to seek the best possible validation of our models. Few techniques are able to yield molecular information about lipids. Recent breakthroughs that break the diffraction-limit barrier are typically only applicable to static structures much larger than a molecular dye. In contrast, lipids are small and dynamic. We briefly discuss below our breakthroughs for comparing neutron scattering with our molecular simulations of complex lipid bilayers.
The projects use the NIH Biowulf computing cluster to run simulations and models. We use molecular dynamics software (such as NAMD and CHARMM) to conduct molecular simulations. In-house software development for public distribution is a key element of the lab's work.
Simple differences in the protein-membrane attachment mechanism have functional consequences for surface mechanics.
We developed two methods for propagating coupled membrane and embedded particle dynamics with ensembles that yield the correct thermodynamics of membrane fluctuations [Reference 1]. Proteins and functional lipids associate with cellular membranes, and their attachments influence the membrane's physical and dynamical properties. Therefore, it is necessary to accurately model the coupled dynamics of the membrane and any associated material of interest. The two methods differ in the binding mechanism of the particle to the surface. The “on-surface” mechanism should be used for particles that slide along the membrane; this description leads to an effective reduction in the membrane surface tension. The “in-surface” mechanism treats the particles as tightly bound to the lipidic binding sites; the method avoids the double-counting of the lateral entropy of implicitly modeled lipids.
The difference between the two attachment mechanisms has significant consequences for crowded membranes in living systems. Local tension is a major factor in determining the ease with which protein machinery can bud off membrane material from the plasma membrane. Likewise, for in vitro experiments on membrane reshaping with ad hoc membrane attachment mechanisms, the presence or lack of additional tension applied is critical for interpreting mechanism.
Efficient computation of protein-lipid interactions in reaction-diffusion modeling
In this study, together with our collaborators in Margaret Johnson's lab, we devised a theoretical treatment of how to properly and efficiently account for proteins interacting with high concentrations of lipids in reaction-diffusion modeling [Reference 2]. Compared with most molecular processes, modeling cellular processes requires large time and length scales. Moreover, the molecules of the cell do not typically move like the objects we interact with in our daily lives. Instead, they move randomly by a diffusive mechanism. Reaction-diffusion modeling uses kinetic data for macromolecular reactions to account for both the slow diffusive motion of molecules as well as for the empirically determined kinetics of their binding and unbinding. In conjunction with our lab's continuum membrane modeling, we are developing a software approach to account for how macromolecular complexes reshape membranes. The study fills an important need for a method that can implicitly model the high concentrations of signaling lipids, such as phosphoinositides, while retaining the rigor and efficiency of reaction diffusion modeling. A critical piece of programming and mathematical analysis was provided by two summer interns in our lab, Ruchita Kothari and Gudrun Thorkelsdottir.
A new mechanism of cholesterol redistribution amongst leaflets of the plasma membrane
In collaboration with Michael Schick and David Allender, we determined the role that curvature stress plays in determining how cholesterol redistributes between the leaflets of the plasma membrane [Reference 3]. A lipid membrane bilayer is made up of two apposed sheets of lipid molecules, oriented so that they form a chemical barrier, which prevents polar material from passively crossing. The inner and outer leaflets of the plasma membrane are composed of very different lipids; the inner is enriched in phosphatidylethanolamine head groups, while the outer leaflet is enriched in sphingolipids. Our lab refined experimental findings on these two lipids, determining that, for example, sphingomyelin prefers significant positive curvature. Cholesterol couples very differently to these lipids' curvature preferences, i.e., it strongly influences the shape of the bilayer around it; thus, around disordered (very fluid) lipids it makes concave leaflets, while around ordered lipids (with the lipid acyl chain tails nearly straight, somewhat gelatinous) it tends to make the surface convex. Our study, which combined simulation and theory, determined that its asymmetric curvature preference prevents cholesterol from accumulating to a high degree in the outer leaflet, where it would otherwise be attracted to the leaflet's saturated lipid content.
Publications
- Sapp K, Maibaum L, Sodt A. Simple differences in the protein-membrane attachment mechanism have functional consequences for surface mechanics. J Chem Phys 2019;151:164116.
- Fu Y, Yogurtcu O, Kothari R, Thorkelsdottir G, Sodt A, Johnson M. An implicit lipid model for efficient reaction-diffusion simulations of protein binding to surfaces of arbitrary topology. J Chem Phys 2019;151:124115.
- Allender D, Sodt A, Schick M. Cholesterol-dependent bending energy is important in cholesterol distribution of the plasma membrane. Biophys J 2019;116:2356-2366.
Collaborators
- David Allender, PhD, Kent State University, Kent State, OH
- Frederick Heberle, PhD, Oak Ridge National Lab, Oak Ridge, TN
- Margaret Johnson, PhD, The Johns Hopkins University, Baltimore, MD
- John Katsaras, PhD, Oak Ridge National Laboratory, Oak Ridge, TN
- Edward Lyman, PhD, University of Delaware, Newark, DE
- Lutz Maibaum, PhD, University of Washington, Seattle, WA
- Michael Schick, PhD, University of Washington in St. Louis, St. Louis, MO
Contact
For more information, email alexander.sodt@nih.gov or visit http://sodtlab.nichd.nih.gov.