Hippocampal Interneurons and Their Role in the Control of Network Excitability
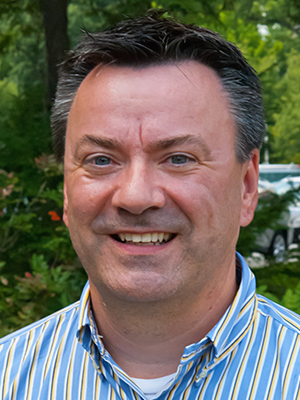
- Chris J. McBain, PhD, Head, Section on Cellular and Synaptic Physiology
- Ramesh Chittajulla, PhD, Staff Scientist
- Kenneth Pelkey, PhD, Staff Scientist
- James D'Amour, PhD, Postdoctoral Fellow
- Vivek Madahavan, PhD, Postdoctoral Fellow
- Jason Wester, PhD, Postdoctoral Fellow
- Steven Hunt, Biologist
- Xiaoqing Yuan, MSc, Biologist
- Carolina Bengtsson, Predoctoral Fellow
- Tyler Ekins, BSc, Predoctoral Fellow
- Connie Mackenzie-Gray Scott, Bsc, Predoctoral Fellow
- Mandy Lai, BSc, Postbaccalaureate Fellow
- Areg Peltekian, BSc, Postbaccalaureate Fellow
Cortical and hippocampal GABAergic inhibitory interneurons (INs) are “tailor-made” to control cellular and network excitability by providing synaptic and extrasynaptic input to their downstream targets via GABAA and GABAB receptors. The axons of this diverse cell population make local, short-range projections (although some subpopulations project their axons over considerable distances) and release the inhibitory neurotransmitter γ-aminobutyric acid (GABA) onto a diverse array of targets. Distinct cohorts of INs regulate sub- and suprathreshold intrinsic conductances, regulate Na+- and Ca2+-dependent action potential generation, modulate synaptic transmission and plasticity, and pace both local- and long-range large-scale synchronous oscillatory activity. An increasing appreciation of the roles played by INs in several neural circuit disorders, such as epilepsy, stroke, Alzheimer’s disease, and schizophrenia, has seen this important cell type take center stage in cortical circuit research. With almost 30 years of interest in this cell type, the main objectives of my lab have been to understand (1) the developmental trajectories taken by specific cohorts of INs as they populate the nascent hippocampus and cortex; (2) how ionic and synaptic mechanisms regulate the activity of both local circuit GABAergic INs and principal neurons (PN) at the level of small, well-defined networks; and (3) how perturbations in their function alter the cortical network in several neural circuit disorders. To this end, we use a variety of electrophysiological, imaging, optogenetic, immunohistochemical, biochemical, molecular, and genetic approaches with both wild-type and transgenic animals.
Neocortical projection neurons instruct inhibitory IN circuit development in a lineage-dependent manner.
For all their complexity, neocortical circuits demonstrate a stereotypical organization across regions, with many repeated motifs, which suggests there are general principles that guide the self-assembly of these circuits during development. A particularly interesting problem for the developing cortex is how to integrate INs, which are produced by progenitors outside the telencephalon, into circuits among locally generated excitatory PNs. Upon reaching the nascent cortex, INs must coordinate with their new host environment to establish proper positioning, wiring, and total numbers. There is accumulating evidence that this process involves subtype-specific interactions between IN and neighboring projection neurons. Investigation of the process is daunting, given the large diversity among INs; however, it is possible that the interactions are organized within primary classes of projection neurons and INs. Recent evidence suggests that the subtype identity of both projection neurons and IN is important for the process.
Jason Wester and Vivek Mahadevan knocked out the transcription factor Satb2 in projection neurons to induce those of the intratelencephalic (IT) type to adopt a pyramidal tract (PT) identity. Loss of IT projection neurons disrupted the lamination and molecular expression profile of INs derived selectively from the caudal ganglionic eminence (CGE) and in a subtype-specific manner. Reprogrammed PNs demonstrated altered connectivity with local CGE INs, with a striking reduction in synaptic targeting of all subtypes. In deep layers of control mice, where IT and PT projection neurons are intermingled, IT projection neurons targeted neighboring CGE INs, while PT projection neurons did not, confirming the lineage-dependent motif. Single-cell RNA sequencing revealed that major CGE IN subtypes were conserved, but with differential transcription of synaptic proteins and signaling molecules. Our data show that both projection neuron class and IN embryonic lineage are important general variables during the construction of cortical circuits.
AMPARs differentially regulate cellular and synaptic maturation of CGE-derived hippocampal interneurons.
In this study, led by former Postdoctoral Fellow Gulcan Akgul, we generated two knockout (KO) mouse lines in which we selectively eliminated Gria2 (GluA2 KO), Gria1, Gria2, and Gria3 (GluA1-3 KO), genes that encode subtypes of ionotropic glutamate receptors, in 5HT3AR–Cre–expressing CGE–derived interneurons. In both the neonatal (postnatal day [P] 5–9) and juvenile (P17–21) hippocampus the frequency of spontaneous excitatory postsynaptic currents (sEPSC) was significantly reduced in GluA2 KO, which was coupled with a reduction in dendritic glutamatergic synapse density. Elimination of GluA1-3 completely eliminated sEPSCs without further reducing synapse density, but increased the complexity of dendritic branching. In GluA1-3 KOs, the number of interneurons invading the hippocampus was increased in the early postnatal period but converged with wild-type (WT) numbers by P21 owing to increased apoptosis. However, GluA1-3 KOs showed an increase in interneurons containing the neurotransmitter CCK and a reduction in those containing the neurotransmitter VIP. Elimination of GluA1-3–containing synapses significantly reduced recruitment of both feedforward and feedback inhibitory input onto pyramidal neurons and altered the contribution of MGE (medial ganglionic eminence)– versus CGE–derived interneuron-mediated inhibition. As a consequence of these combined anatomical, synaptic, and circuit alterations, GluA2 KO and GluA1 KO mice exhibited impaired learning and deficits in social behavior. Thus, AMPAR subunits differentially contribute to numerous aspects of the development and maturation of CGE–derived interneurons and hippocampal circuitry that are essential for normal animal behavior.
Shisa7, a GABAA receptor auxiliary subunit controlling benzodiazepine actions
GABA type A receptors (GABAARs) mediate fast inhibitory transmission in the mammalian brain and are ligand-gated pentameric anion channels assembled from various combinations of 19 subunits. The abundance and kinetics of GABAARs at synapses fundamentally control inhibitory synapse strength and neural circuit information processing. Whether native GABAARs contain additional auxiliary subunits that control both trafficking and kinetics of the receptor remains unknown. GABAARs are also the primary targets for several drugs, notably benzodiazepines (BDZs), barbiturates, anesthetics, and ethanol. The function and pharmacology of GABAARs are of great physiological and clinical importance and have long been thought to be determined by the channel pore–forming subunits. In a collaboration with the lab of Wei Lu, we discovered that Shisa7, a single-passing transmembrane protein, localizes at GABAergic inhibitory synapses and interacts with GABAARs. Shisa7 controls receptor abundance at synapses and accelerates the channel deactivation kinetics. Shisa7 also potently enhances the action of diazepam, a classic benzodiazepine, on GABAARs. Genetic deletion of Shisa7 selectively impairs GABAergic transmission and diminishes the effects of diazepam in mice. Our data indicate that Shisa7 regulates GABAAR trafficking, function, and pharmacology and reveal a previously unknown molecular interaction that modulates benzodiazepine action in the brain.
Circuit malformation and rearrangement in a mouse model of classic type I lissencephaly
Classical lissencephaly is a rare neurodevelopmental disease caused by haploinsufficiency of the LIS1 gene and is characterized in humans by brain malformation, developmental delays, and hyperexcitability. The deficits are recapitulated in a mouse model of lissencephaly. As Lis1 protein is required for cellular migration, we used this model to investigate the impact of disrupted neuronal migration on both PN and IN microcircuit formation.
PNs of the developing mouse hippocampus arise in proliferative waves of cell birth and migration between E12–17. Later, PNs appear as a densely packed monolayer of neurons, in a principal cell layer (PCL). Emerging evidence suggests the existence of many excitatory cell subtypes within the PCL. James (Jim) D’amour is investigating two populations of PNs readily identified in the superficial-deep axis of the hippocampus: calbindin (CB)-positive and calbindin-negative PNs. CB immunostaining labels superficial PNs of the PCL, while the deeper half of the PCL is largely devoid of CB expression. In normal animals, CB expression confers a complex dendritic morphology, sensitivity to endocannabinoids, greater sag current conductance, and preferential targeting of IN subtypes. These data suggest that CB–expressing PCs form a previously underappreciated layer within the PCL, akin to the layers within the neocortex, but compressed into a single band within the evolutionarily older hippocampus. Jim next studied this CB subpopulation of cells in the mouse model of lissencephaly. Mice heterozygous for the Lis1 gene display fractured PCLs into deep and superficial bands. He found that calbindin-expressing PNs are preferentially located in the deeper band (opposite of their normal superficial positioning) and is currently probing the interplay between cellular positioning, genetic identity, and cellular birthdate in the formation of brain circuits. Preliminary data suggest that ectopically positioned CB–PNs retain their intrinsic properties, including morphology and ion channel expression. However, connectivity to extrinsic afferents and local IN subpopulations appear to be perturbed, altering the role played by these PNs in generating coherent oscillations.
In a second related study, Tyler Ekins characterized the distributions of INs in the Lis1+/– CA1 hippocampus. Although the total density of IN classes (PV-, SOM-, RN-, CCK-) reach the hippocampus in normal quantities, PV–containing INs migrate towards more superficial regions and are found in ectopic positions deep in stratum radiatum in the Lis1+/– mouse. He next eliminated one copy of LIS1 from specific cell types: Emx-Cre (Lis1 removed from PNs), Nkx2.1-Cre (Lis1 removed from MGE–derived INs). Interestingly, Emx-Cre and Nkx-Cre lines resulted in a pattern similar to the global Lis1+/– line: no change in overall cell density, but shift PV INs towards superficial regions of the CA1, indicating both cell-autonomous and non-autonomous roles for Lis1 in IN migration. Cluster analysis of PV–IN electrophysiological properties identified two subpopulations: fast-Spiking (FS) with higher rheobase and firing frequency and lower input resistance, and fast-spiking-like (FSL) cells with lower rheobase and firing frequency and higher input resistance. FS cells consist of the standard subtypes (basket, bistratified, and axo-axonic cells), but FSL cells had atypical PV cell morphologies and, in WT, represent a minor population. In contrast, although Lis1+/– PV cells also sorted into clusters of FS and FSL cells, FSL cells were the dominant population, particularly among cells positioned in the stratum radiatum. He next recorded the output of PV INs and found that basket cells provide stronger inhibition to morphologically defined “simple” PNs (see above), which tend to occupy the deeper half of the PCL than “complex” superficially located PNs. This pattern held true in the LIS1 KO, even among PNs in ectopic positions. Synaptically coupled PV and PN pairs demonstrate that unitary inhibitory connections from WT basket cells are strongest on simple PNs than complex PNs. Further paired recordings will elucidate the connectivity patterns and dynamics of FS/FSL cells in the LIS hippocampus and help us gain a better understanding of how cellular migration drives synaptogenesis and circuit formation.
Additional Funding
- Jason Wester was funded by an NINDS Intramural National Research Service Award (NRSA).
- James D’Amour was funded by an NIGMS PRAT Fellowship.
Publications
- Pelkey KA, Chittajallu R, Craig MT, Tricoire L, Wester JC, McBain CJ. Hippocampal GABAergic inhibitory interneurons. Physiol Rev 2017;97:1619-1747.
- Han W, Li J, Pelkey KA, Pandey S, Chen X, Wang Y, Wu K, Ge L, Tianming L, Castellano D, Liu C, Wu L-G, Petralia RS, Lynch JW, McBain CJ, Lu W. Shisa7 is a GABAA receptor auxiliary subunit controlling benzodiazepine actions. Science 2019;366:246-250.
- Wester J, Mahadevan V, Rhodes C, Calvigioni D, Venkatesh S, Maric D, Hunt S, Yuan X-Q, Zhang Y, Petros TJ, McBain CJ. Neocortical projection neurons instruct inhibitory interneuron circuit development in a lineage dependent manner. Neuron 2019;102:960-975.
- Akgul G, Abebe D, Yuan X-Q, Auville K, McBain CJ. The role of AMPARs in the maturation and integration of caudal ganglionic eminence-derived interneurons in developing hippocampal circuits. Sci Rep 2019;9:5435.
Collaborators
- Wei Lu, PhD, Synapse and Neural Circuit Unit, NINDS, Bethesda, MD
- Timothy J. Petros, PhD, Unit on Cellular and Molecular Neurodevelopment, NICHD, Bethesda, MD
Contact
For more information, email mcbainc@mail.nih.gov or visit https://www.nichd.nih.gov/research/atNICHD/Investigators/mcbain or https://neuroscience.nih.gov/Faculty/Profile/chris-mcbain.aspx.