Genomics of Development in Drosophila melanogaster
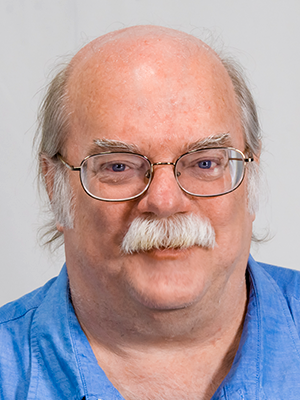
- James A. Kennison, PhD, Head, Section on Drosophila Gene Regulation*
- Monica T. Cooper, BA, Senior Research Technician
* Dr. Kennison has retired as of January 1, 2020.
Our goal is to understand how linear information encoded in genomic DNA controls cell fates during development. The Drosophila genome is about one twentieth the size of the human genome. However, despite its smaller size, most developmental genes and at least half of the disease- and cancer-causing genes in humans are conserved in Drosophila, making Drosophila an excellent model system for the study of human development and disease. One of the important groups of conserved developmental genes are the homeotic genes. In Drosophila, the homeotic genes specify cell identities at both embryonic and adult stages. The genes encode homeodomain-containing transcription factors that control cell fates by regulating the transcription of downstream target genes. The homeotic genes are expressed in precise spatial patterns that are crucial for the proper determination of cell fate. Both loss of expression and ectopic expression in the wrong tissues lead to changes in cell fate. The changes provide powerful assays for identifying the trans-acting factors that regulate the homeotic genes and the cis-acting sequences through which they act. The trans-acting factors are also conserved between Drosophila and human and have important functions, not only in development but also in stem-cell maintenance and cancer.
Cis-acting sequences for transcriptional regulation of the Sex combs reduced (Scr) homeotic gene
Assays in transgenes in Drosophila previously identified cis-acting transcriptional regulatory elements from homeotic genes. The assays found tissue-specific enhancer elements as well as cis-regulatory elements that are required for the maintenance of activation or repression throughout development. While these transgenic assays have been important in defining the structure of the cis-regulatory elements and identifying trans-acting factors that bind to them, their functions within the context of the endogenous genes remain poorly understood. We used a large number of existing chromosomal aberrations in the Scr homeotic gene to investigate the functions of the cis-acting elements within the endogenous gene. The chromosomal aberrations identified an imaginal leg enhancer about 35 kb upstream of the Scr promoter. The enhancer is not only able to activate transcription of the Scr promoter that is 35 kb distant but can also activate transcription of the Scr promoter on the homologous chromosome. Although the imaginal leg enhancer can activate transcription in all three pairs of legs, it is normally silenced in the second and third pairs of legs. The silencing requires the Polycomb-group proteins. We are currently attempting to identify the cis-regulatory DNA sequences in the Scr that are required for Polycomb-group silencing in the second and third legs. Characterization of the chromosomal rearrangements shown in Figure 1 also revealed that two genetic elements (proximal and distal MES [maintenance elements for silencing]), about 70 kb apart in the Scr gene, must be in cis to maintain proper repression. When not physically linked, the elements interact with elements on the homologous chromosome and cause derepression of its wild-type Scr gene. Using a transgenic assay, we identified at least five DNA fragments from the Scr gene that silence transcription from a reporter gene. The transcriptional silencers are clustered in the two regions whose interactions are required for the maintenance of silencing in the endogenous genes. We used the Crisper/Cas9 methodology to generate chromosomes lacking one, two, or three of the silencing elements. Silencing is only disrupted when multiple elements are deleted.
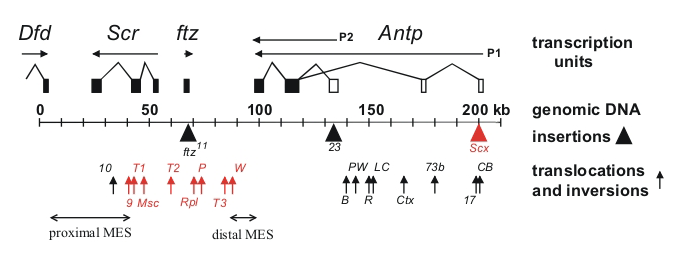
Click image to view.
Figure 1. Chromosomal aberrations in the distal half of the Antennapedia complex
The transcription units are shown above the genomic DNA, while chromosomal aberrations are shown below (solid triangles indicate insertions of transposable elements and upward arrows indicate breakpoints of translocations and inversions). Chromosomal aberrations (red) interfere with silencing in the adult second and third legs. The regions that include the proximal and distal MES are indicated by horizontal arrows.
Trans-acting activators and repressors of homeotic genes
The initial domains of homeotic gene repression are set by the segmentation proteins, which also divide the embryo into segments. Genetic studies identified the trithorax group of genes that are required for expression or function (such as maintenance of transcriptional activation) of the homeotic genes. Maintenance of transcriptional repression requires the proteins encoded by the Polycomb-group genes. To identify new trithorax-group activators and Polycomb-group repressors, we screened for new mutations that mimic the following phenotypes: loss of function or ectopic expression of the homeotic genes. We generated over 4,000 lethal mutants and, among those that die late in development, identified two dozen mutants with homeotic phenotypes. Some of the homeotic phenotypes are shown in Figure 2. The mutants identify genes required for expression or function of the homeotic genes.
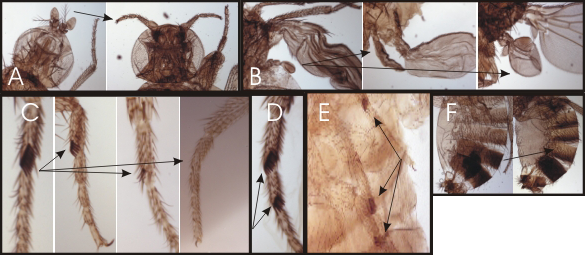
Click image to view.
Figure 2. Homeotic phenotypes of new pharate-adult lethal mutants
A. Wild-type on the left and the transformation of aristae to distal leg on the right.
B. Wild-type haltere on the left and transformations of anterior and posterior haltere to anterior and posterior wing in the middle and right, respectively.
C. First legs from a wild-type male on the left and three different mutants with reduced sex combs on the right.
D. Mutant male with sex combs on both the first and second tarsal segments.
E. Mutant male with sex combs on all three pairs of legs.
F. Abdominal segments from a wild-type male on the left; mutant male with transformation of the fifth abdominal segment to a more anterior identity on the right.
We also use Polycomb-group response elements from the Scr gene to screen for recessive Polycomb-group mutations. Transgenes with a Polycomb-group response element and a reporter gene (the Drosophila mini-white gene) exhibit reporter gene expression in flies heterozygous for the transgene, but reporter gene expression is repressed in flies homozygous for the transgene. In flies homozygous for transgenes with the mini-white reporter gene silenced by the Polycomb-group response elements, we generate clones of cells in the eye that are homozygous for newly induced mutations, using the yeast FLP/FRT site-specific recombination system (Figure 3). Silencing mutations are detected by the appearance of pigmented spots in the white-eyed flies (cells that derepress the silenced mini-white reporter gene). We screened about 98% of the genome and recovered 343 new silencing mutants. About one third of the new mutants do not carry a new silencing mutation but bear chromosome aberrations that generate aneuploid cells after mitotic recombination. The aneuploid regions include the reporter transgene, and they disrupt silencing by changing copy number. Although the mutants do not identify new genes, the phenomenon that we discovered will be very useful for detecting chromosomal aberrations in F1 mutant screens. The remaining two thirds of our mutants are not associated with large chromosomal aberrations and carry mutations in genes required for Polycomb-group transcriptional silencing. Seventy-seven mutations are in 15 known Polycomb-group genes (Pc, ph-d, ph-p, Psc, Su(z)2, Sce, E(z), Su(z)12, esc, Sfmbt, Asx, calypso, Pcl, Scm, and crm). The remaining mutations identify 45 additional genes required for silencing. For 31 of the silencing genes, we identified the corresponding transcription units using a combination of meiotic-recombination mapping and whole-genome sequencing. The new silencing genes encode DNA–binding proteins (Spps, ftz-f1, grh, Dsp1, cut, Nf-YB, CG17829, and ocm), chromatin-remodeling factors (Mi-2, Iswi, and CG16908), chromatin-modifying and chromatin-associated factors (gpp, Tip60, CG9293, CG43736, and ptip), insulator proteins, and two aminoacyl-tRNA synthetases (ThrRS and AsnRS).
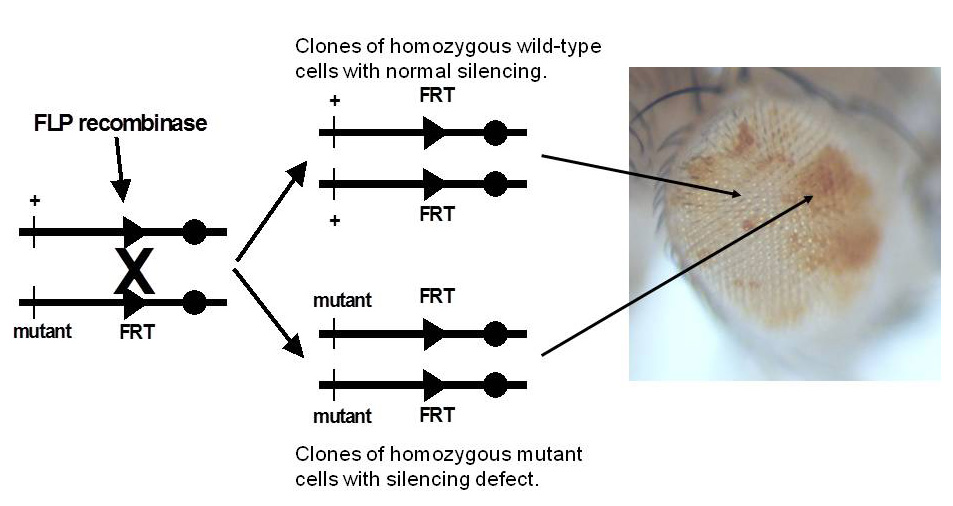
Click image to view.
Figure 3. Genetic screen for new mutations that disrupt pairing-sensitive silencing
Flies homozygous for transposons carrying the mini-white reporter gene and a pairing-sensitive silencing element have white eyes. Clones of cells homozygous for newly induced mutants are generated using the yeast site-specific recombinase (FLP recombinase) and its target site (FRT). The clones of mutant cells are able to express the mini-white reporter gene and are pigmented (shown in the eye on the right of the figure).
Structure and function of the Drosophila genome
The Drosophila melanogaster genome has been intensely studied for over 100 years. Recently, sequencing of the majority of the genomic DNA revealed much about the structure and organization of the genome. Despite those molecular advances, much remains to be discovered about the functions encoded within the genome. In addition to transcriptional regulation exerted through cis-regulatory elements by trans-acting factors, gene regulation can also be influenced by the gene's location in the genome. Regulation of gene expression at the chromosomal level is a common phenomenon, and several examples have been extensively characterized, such as X chromosome inactivation in female mammals and hypertranscription of X chromosome genes in male Drosophila. Less well-known examples are silencing or loss of paternally inherited chromosomes in male coccids and the unusual chromosomal behavior at several stages in the life cycle of the fungus fly Sciara coprophila. It was the investigations into chromosome behavior in Sciara that first used the term “imprinting” to describe the ability of some organisms to recognize the parental origin of their genetic material.
An important tool in discovering or investigating many examples of chromosomal regulation has been the production of translocations, which exchange portions of chromosomes. We are investigating another example of chromosomal regulation that affects spermatogenesis in both Drosophila and mammals. Translocations between sex chromosomes and autosomes disrupt male fertility. The basis for the disruption is still not well understood. About two-thirds of X-autosome translocations in Drosophila are male-sterile, a sterility that is dominant and male-specific. The X chromosome breakpoints of the male-fertile X-autosome translocations are not random; the nonrandom pattern led investigators 50 years ago to propose a model in which the X chromosome is precociously inactivated during spermatogenesis and in which X-autosome translocations disrupt this precocious X inactivation. The major roadblock to testing this model is that only one mutant strain with a male-sterile X-autosome translocation is currently available, as all other X-autosome mutant strains were lost decades ago. We generated a new collection of 80 X-autosome translocations to investigate the molecular basis of the dominant male sterility caused by translocations. We are using these translocations to test whether the disruption of male fertility is attributable to transcriptional defects (as first proposed almost fifty years ago) or to defects in chromatin remodeling or in condensation for packaging into the sperm head.
Publications
- Monribot-Villanueva J, Juarez-Uribe RA, Palomera-Sanchez Z, Gutierrez-Aguiar L, Zurita M, Kennison JA, Vazquez M. TnaA, an SP-RING protein, interacts with Osa, a subunit of the chromatin remodeling complex BRAHMA and with the SUMO pathway in Drosophila melanogaster. PLoS One 2013 8:e62251.
- Lindsley DL, Roote J, Kennison JA. Anent the genomics of spermatogenesis in Drosophila melanogaster. PLoS One 2013 8:e55915.
- Gilliland WD, May DP, Colwell EM, Kennison JA. A simplified strategy for introducing genetic variants into Drosophila compound autosome stocks. G3 2016 6:3749-3755.
- Kassis JA, Kennison JA, Tamkun JW. Polycomb and trithorax group genes in Drosophila. Genetics 2017 206:1699-1725.
Collaborators
- William Gilliland, PhD, DePaul University, Chicago, IL
- Judith Kassis, PhD, Section on Gene Expression, NICHD, Bethesda, MD
- Dan Lindsley, PhD, University of California San Diego, La Jolla, CA
- Brian Oliver, PhD, Laboratory of Cellular and Developmental Biology, NIDDK, Bethesda, MD
- Martha Vazquez, PhD, Instituto de Biotecnología, UNAM, Cuernavaca, Mexico
- Mario Zurita, PhD, Instituto de Biotecnología, UNAM, Cuernavaca, Mexico
Contact
For more information, email james.kennison@nih.gov.