Thyroid Hormone Regulation of Vertebrate Postembryonic Development

- Yun-Bo Shi, PhD, Head, Section on Molecular Morphogenesis
- Liezhen Fu, PhD, Staff Scientist
- Nga Luu, MS, Biologist
- Lusha Liu, PhD, Visiting Professor
- Keisuke Nakajima, PhD, Visiting Professor
- Lu Xue, PhD, Visiting Professor
- Wonho Na, PhD, Visiting Fellow
- Yuki Shibata, PhD, Visiting Fellow
- Yuta Tanizaki, PhD, Visiting Fellow
- Lingyu Bao, MS, Graduate Student
- Shouhong Wang, BS, Graduate Student
- Robert Liu, BS, Postbaccalaureate Fellow
This laboratory investigates the molecular mechanisms of thyroid hormone (TH) function during postembryonic development, a period around birth in mammals when plasma levels of TH peak. The main model we use is the metamorphosis of Xenopus laevis and X. tropicalis, two highly related species that offer unique but complementary advantages. The control of this developmental process by TH offers a paradigm to study gene function in postembryonic organ development. During metamorphosis, different organs undergo vastly different changes. Some, like the tail, undergo complete resorption, while others, such as the limb, are developed de novo. The majority of the larval organs persist through metamorphosis but are dramatically remodeled to function in a frog. For example, tadpole intestine is a simple tubular structure consisting primarily of a single layer of larval epithelial cells. During metamorphosis, through specific larval epithelial cell death and de novo development of the adult epithelial stem cells, followed by their proliferation and differentiation, the intestine is transformed into an organ with a multiply folded adult epithelium surrounded by elaborate connective tissue and muscles. The wealth of knowledge from past research and the ability to manipulate amphibian metamorphosis both in vivo, using genetic approaches or hormone treatment of whole animals, and in vitro in organ cultures offer an excellent opportunity to (1) study the developmental function of TH receptors (TRs) and the underlying mechanisms in vivo and (2) identify and functionally characterize genes that are critical for organogenesis, particularly the formation of the adult intestinal epithelial stem cells, during postembryonic development in vertebrates. A major recent focus has been to make use of the TALEN and CRISPR/Cas9 technologies to knockdown or knockout the endogenous genes to permit functional analyses. In addition, we complement our frog studies by investigating the genes found to be important for frog intestinal stem cell development in developing mouse intestine by making conditional knockouts.
Unique role of TRβ in regulating notochord resorption during Xenopus metamorphosis
Tail resorption during anuran metamorphosis is perhaps the most dramatic tissue transformation that occurs during vertebrate development. Like all other process during metamorphosis, tail resorption is controlled by TH. Earlier studies in the anuran species X. laevis and X. tropicalis had shown that the TR plays a necessary and essential role for metamorphosis. Of the two known TR genes in all vertebrates, trα is highly expressed during both premetamorphosis and metamorphosis, while trβ expression is low in premetamorphic tadpoles but highly upregulated as a direct target gene of TH during metamorphosis. In addition, the two TR genes have distinct temporal regulation patterns in different organs during development, suggesting that the two TRs have different functions during metamorphosis. Indeed, gene knockout studies by us and others showed that trα is not essential for metamorphosis but controls metamorphic timing and the rate of metamorphosis progression during early metamorphosis. Knockout of trβ, however, has no effect on metamorphic timing or early metamorphosis, but significantly delays late metamorphosis, particularly tail resorption. Homozygous trβ knockout tadpoles become tailed frogs well after their wild-type siblings complete metamorphosis. Most noticeably, in trβ-knockout tadpoles, an apparently normal notochord is present in the tail as late as three days after the initiation of tail shortening (stage 62), while in wild-type and trα-knockout tadpoles, the tail notochord disappears in about one day. We investigated how tail notochord resorption is regulated by TR [Reference 1]. We showed that trβ is selectively much more highly expressed in the notochord than is trα.
We also discovered differential regulation of several matrix metalloproteinases (MMPs), which are known to be upregulated by TH and thought to play a role in tissue resorption by degrading the extracellular matrix (ECM). In particular, MMP9-TH and MMP13 are extremely highly expressed in the notochord compared with the rest of the tail. In situ hybridization analyses show that these MMPs are expressed in the outer sheath cells and/or the connective tissue sheath surrounding the notochord. Our findings suggest that high levels of trβ expression in the notochord specifically upregulate the MMPs, which in turn degrades the ECM, leading to the collapse of the notochord and its subsequent resorption during metamorphosis.
Gene expression program underlying tail resorption during thyroid hormone–dependent metamorphosis of the ornamented pygmy frog Microhyla fissipes
Studies on amphibian metamorphosis have largely focused on the two highly related species X. laevis and X. tropicalis. Adult X. laevis and X. tropicalis animals remain aquatic, in contrast to most other anurans, which change from aquatic to terrestrial during metamorphosis, and thus more closely mimic the postembryonic development in mammals, making it important to study metamorphosis in a truly terrestrial frog species. In this regard, the anuran Microhyla fissipes offers several advantages as an alternative model for developmental and genetic studies. We made use of the advances in sequencing technologies to investigate the gene regulation profiles underlying the tail resorption program during metamorphosis in M. fissipes [Reference 2]. We first used single-molecule real-time (SMRT) sequencing to obtain 67,939 expressed transcripts in M. fissipes. We next identified 4,555 differentially expressed transcripts (DETs) during tail resorption by using Illumina sequencing on RNA samples from tails at different metamorphic stages. Bioinformatics analyses revealed that several KEGG (Kyoto Encyclopedia of Genes and Genomes) pathways and GO (Gene Ontology) terms associated with tail resorption were enriched. Our findings suggest that tail resorption during M. fissipes and X. laevis shares many of the same programs. Future investigations into the function and regulation of these genes and pathways should help to reveal the mechanisms governing amphibian tail resorption and adaptive evolution from aquatic to terrestrial life. Furthermore, analysis of the M. fissipes model, with particular regard to the changes in other organs associated with the transition from aquatic to terrestrial living, should help to yield important mechanistic insights into mammalian postembryonic developments.
TH activates the Xenopus mbd3 gene via an intronic TRE during intestinal stem cell development.
To identify direct TH–response genes during the formation of adult intestinal stem cells, we previously carried out a ChIP (chromatin immunoprecipitation)-on-chip analysis with a polyclonal anti-TR antibody on the tadpole intestine and identified many putative TR target genes. Among them is the methyl-CpG binding domain protein 3 (mbd3) gene, which has been implicated in epigenetic regulation of cellular processes as a subunit of the Mi-2/NuRD (Nucleosome Remodeling Deacetylase) complex. We showed that mbd3 is upregulated in the intestine by TH and that its expression peaks at stage 62, the climax of metamorphosis [Reference 3]. We further discovered a putative thyroid hormone response element (TRE) within the first intron of the mbd3 gene that binds to TR/RXR in vitro and in vivo and mediates TH regulation of the mbd3 promoter in vivo.
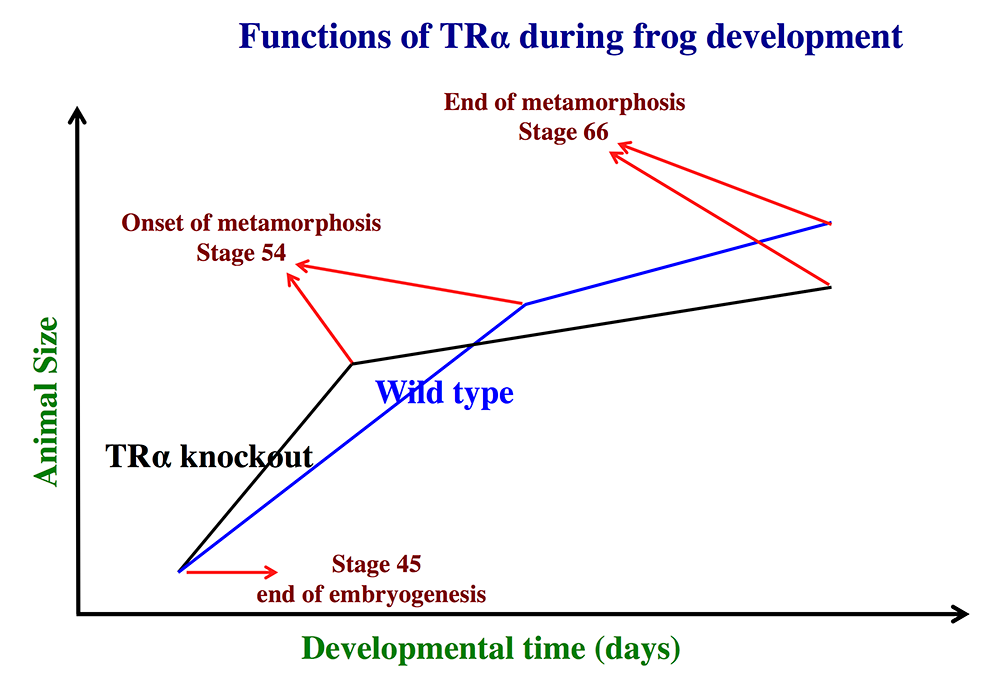
Click image to view.
Figure 1. Schematics showing the effects of TRα (thyroid hormone receptor α) knockout on Xenopus tropicalis development
TRα knockout has little effect on embryogenesis, and resulting tadpoles are normal by feeding stage (stage 45). However, once feeding begins, the animals grow at different rates, with the knockouts growing faster; they are thus larger than wild-type siblings at the same age (in days) (comparing the vertical axis values of the lines for the knockout and wild-type animals at any given position along the horizontal axis between stages 45 and 54). The knockout animals also develop faster, reaching developmentally more advanced stages than wild-type siblings at the same age (in days). Thus, the knockout animals reach stage 54, the onset of metamorphosis, at a younger age (see the horizontal axis locations for the upper end of the lines). Interestingly, when the animals are compared at stage 54, the wild-type are larger than the knockout siblings, even though the latter grow faster. This is because the wild-type animals take longer to reach metamorphosis (stage 54). The extra growth time needed to reach stage 54 enables the wild-types to catch up and surpass the knockouts in size. After the initiation of metamorphosis at stage 54, the knockout tadpoles metamorphose more slowly than the wild-type ones, enabling the latter to catch up in development, with both groups finishing metamorphosis at around the same age. The knockout animals initiate metamorphosis at a smaller size and also end up smaller at the end of metamorphosis than do the wild-type siblings. Thus, in premetamorphic tadpoles prior to stage 54, unliganded TRα (due to the lack of thyroid hormone) functions to control metamorphic timing, whereas, when thyroid hormone becomes available during metamorphosis, TRα helps increase the rate of metamorphosis.
TRα mutations lead to epithelial defects in the adult intestine in a mouse model of resistance to thyroid hormone.
Intestinal maturation in mammals takes place around birth when TH levels are high, mimicking frog metamorphosis and implicating a role of TH in mammalian intestinal development and function. Interestingly, a several human patients carrying heterozygous mutations in the TRα gene (RTHα, or resistance to TH due to TRα mutation) have been discovered in recent years and found to suffer from constipation, implicating intestinal defects caused by TRα gene mutations. To determine how TRα1 mutations affect the intestine, we analyzed a mutant mouse expressing a strong dominantly negative TRα1 mutant, (denoted TRα1PV; Thra1PV mice) [Reference 4]. The mutant mouse faithfully reproduces RTHα phenotypes observed in human patients. In adult Thra1PV/+ mice, we observed constipation just like in patients with TRα mutations. Importantly, we discovered significant intestinal defects, including shorter villi and higher numbers of differentiated cells in the crypt, accompanied by reduced stem-cell proliferation in the intestine. Our findings suggest an evolutionary conservation of TH function in the intestine. Further analysis of this mouse model should help reveal the molecular and physiological defects in the intestine caused by TRα mutations and determine the underlying mechanisms.

Click image to view.
Figure 2. Intestinal metamorphosis involves the formation of clusters of proliferating, undifferentiated epithelial cells at the climax.
Tadpoles at premetamorphic stage 54 (A), climax (B, stage 62), and end of metamorphosis (C, stage 66) were injected with 5-ethynyl-2′-deoxyuridine (EdU) one hour before being sacrificed. Cross-sections of the intestine from the resulting tadpoles were double-stained by EdU labeling of newly synthesized DNA and by immunohistochemistry of IFABP (intestinal fatty acid–binding protein), a marker for differentiated epithelial cells. The dotted lines depict the epithelium-mesenchyme boundary. Note that there are few EdU–labeled proliferating cells in the epithelium and that they express IFABP at premetamorphosis (A) and increase in the form of clustered cells (proliferating adult stem cells), which lack IFABP at the climax of metamorphosis (B). At the end of metamorphosis, EdU–labeled proliferating cells are localized mainly in the troughs of the epithelial folds, where IFABP expression is low (C). ep: epithelium; ct: connective tissue; m: muscles; l: lumen.
LAT1 regulates osteoclastogenesis and bone homeostasis through the mTORC1 pathway.
To regulate cellular processes, TH has to be actively transported into cells, a process that is mediated by several different types of transporters. LAT1, one of our previously identified TH–response genes in the intestine, encodes the light chain of a heterodimeric system L type of TH transporter, which also transports several amino acids. Interestingly, Lat1 is highly upregulated at the climax of metamorphosis in the tadpole intestine, coinciding with the formation and rapid proliferation of adult intestinal stem cells. We also found out that LAT1 was also highly expressed in the mouse intestine during the neonatal period when the mouse intestine matures into the adult form, a process that appears also involves TH–dependent formation and/proliferation of the adult intestinal stem cells. In a collaborative study, we generated a mouse line with the Lat1 gene floxed, which allows conditional knockout of the Lat1 upon expression of the Cre recombinase. We are still in the process investigating whether LAT1 affects adult intestinal stem-cell development in mouse, but we recently showed, through another collaboration, that LAT1 is an important amino acid transporter for the regulation of bone homeostasis through its function in osteoclasts [Reference 5]. Lat1 expression was significantly reduced in osteoclasts in a mouse model of ovariectomy-induced osteoporosis. The osteoclast-specific deletion of Lat1 in mice led to osteoclast activation and bone loss in vivo, and LAT1 deficiency elevated osteoclastogenesis in vitro. Loss of LAT1 impaired activation of the mechanistic target of rapamycin complex 1 (mTORC1) pathway in osteoclasts, whereas genetic activation of mTORC1 corrected the activation of osteoclastogenesis and bone loss resulting from LAT1 deficiency. The findings suggest that the LAT1–mTORC1 axis plays a pivotal role in bone resorption and bone homeostasis, thereby providing a novel molecular connection between amino-acid intake and skeletal integrity.
Additional Funding
- Japan Society for the Promotion of Science (JSPS) fellowships for Drs. Yuta Tanizaki and Yuki Shibata
- Awards from the China Scholarship Council (CSC) to Drs. Shouhong Wang, Lu Xue, and Lusha Liu
- Keisuke Nakajima was supported by Hiroshima University, Japan
Publications
- Nakajima K, Tazawa I, Shi Y-B. A unique role of thyroid hormone receptor ß in regulating notochord resorption during Xenopus metamorphosis. Gene Comp Endocrinol 2019;277:66-72.
- Wang S, Liu L, Liu J, Zhu W, Tanizaki Y, Fu L, Bao L, Shi Y-B, Jiang J. Gene expression program underlying tail resorption during thyroid hormone-dependent metamorphosis of the ornamented pygmy frog Microhyla fissipes. Front Endocrinol (Lausanne) 2019;10:11.
- Fu L, Li C, Na W, Shi, Y-B. Thyroid hormone activates Xenopus MBD3 gene via an intronic TRE in vivo. Front Biosci (Landmark Ed) 2020;25:437-451.
- Bao L, Roediger J, Park S, Fu L, Shi B, Cheng S-Y, Shi, Y-B. Thyroid hormone receptor alpha mutations lead to epithelial defects in the adult intestine in a mouse model of resistance to thyroid hormone. Thyroid 2019;29(3):439-448.
- Ozaki K, Yamada T, Horie T, Ishizaki A, Hiraiwa M, Iezaki T, Park G, Fukasawa K, Kamada H, Kaneda K, Ogawa K, Ochi H, Sato S, Kobayashi Y, Shi Y-B, Taylor PM, Hinoi E. The L-type amino acid transporter LAT1 inhibits osteoclastogenesis and maintains bone homeostasis through the mTORC1 pathway. Sci Signal 2019;12(589):pii: eaaw3921.
Collaborators
- Sheue-Yann Cheng, PhD, Laboratory of Molecular Biology, NCI, Bethesda, MD
- Jianping Jiang, PhD, Chengdu Institute of Biology, Chinese Academy of Sciences, Chengdu, China
- Eiichi Hinoi, PhD, Kanazawa University Graduate School, Kanazawa, Japan
- Bingyin Shi, MD, Xi’an Jiaotong University School of Medicine, Xi'an, China
- Guihong Sun, PhD, Wuhan University School of Medicine, Wuhan, China
- Peter Taylor, PhD, University of Dundee, Dundee, UK
- Ichiro Tazawa, PhD, Amphibian Research Center, Hiroshima University, Japan
Contact
For more information, email shi@helix.nih.gov or visit http://smm.nichd.nih.gov.