Biophysics of Large Membrane Channels
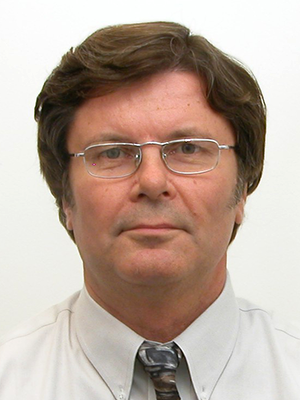
- Sergey M. Bezrukov, PhD, DSci, Head, Section on Molecular Transport
- Philip A. Gurnev, PhD, Staff Scientist
- Tatiana K. Rostovtseva, PhD, Staff Scientist
- Amandine Rovini, PhD, Visiting Fellow
We study mitochondrial and bacterial membrane proteins that form the “large” beta-barrel channels responsible for metabolite fluxes between cells and cellular compartments. In particular, we are interested in the physical mechanisms of their functioning and regulation under normal and pathological conditions. Among many wet-lab approaches, such as fluorescence correlation spectroscopy, bilayer overtone analysis, and confocal microscopy, our hallmark method is reconstitution of these proteins into planar lipid membranes in order to study them at the single-molecule level. Empirical findings obtained in these experiments are rationalized within the framework of a physical theory of channel-facilitated transport, an understanding that is necessary to design new strategies to effectively correct the deviant interactions associated with disease.
In contrast to the highly ion-selective channels studied in neurophysiology, which have narrow selectivity filters to match the size of a partially dehydrated ion, metabolite or “nutrient” channels are significantly wider. Indeed, they have to accommodate metabolite molecules that are typically much larger than simple mono- or divalent ions. Because of their size and primarily beta-barrel scaffolds, the mechanisms of the selectivity and gating of these channels are quite different from those of the highly ion-selective channels formed from alpha-helical subunits. To grasp the general principles of beta-barrel channel functioning and regulation, we work with a variety of proteins and channel-forming peptides. Among them are VDAC (voltage-dependent anion channel from the outer membrane of mitochondria), alpha-Hemolysin (toxin from Staphylococcus aureus), translocation pores of Bacillus anthracis (PA63), Clostridium botulinum (C2IIa) and Clostridium perfringens (Ib) binary toxins, Epsilon toxin (from Clostridium perfringens), OmpF (general bacterial porin from Escherichia coli), LamB (sugar-specific bacterial porin from Escherichia coli), OprF (porin from Pseudomonas aeruginosa), Syringomycin E (lipopeptide toxin from Pseudomonas syringae), and the bacterial peptide TisB, which is involved in persister cell biofilm formation. We also use Gramicidin A (linear pentadecapeptide from Bacillus brevis) as a molecular sensor of membrane-mechanical properties. To study the channel-forming proteins under controlled conditions, we first isolate them from their host organisms, purify them, and then reconstitute them into planar lipid bilayers, a model system with precisely defined physical properties. This allows us to explore channel interactions with the lipid membrane as modified by volatile anesthetics, by cytosolic proteins, such as tubulin and alpha-synuclein, and by newly synthesized drugs, such as blockers of the translocation pores of bacterial toxins. Our motivation is that learning the molecular mechanisms of channel functioning is vital for developing new approaches to treatment of various diseases for which regulation of transport through ion channels plays the key role.
Partitioning of soft water-soluble polymers into beta-barrel channels in their functional states

Click image to enlarge.
Figure 1. Polymers pushing polymers: the effect of differently sized PEGs and their mixtures on channel conductance (Reference 1)
Traces labeled “No PEG” show the current jump immediately after spontaneous channel formation. Addition of 15% (wt/wt) PEG200 causes a significant drop in single-channel conductance: 30% in case of VDAC; 45% in case of alpha-Hemolysin (aHL); and 35% in case of OmpC. Addition of 15% (wt/wt) PEG 3400, which does not penetrate the channel at this concentration, causes an apparent increase in single-channel conductance (VDAC, 2%; aHL, 10%; and OmpC, 2%). Addition of 15% (wt/wt) PEG200 along with 15% (wt/wt) of PEG3400 reduces the channel conductance by an extra 15% as a result of additional PEG200 partitioning into the pore, as it is “pushed” by PEG3400 molecules in the bathing solution. Current jumps after PEG addition correspond to the moments of transmembrane voltage application of 30 mV.
The cell is a crowded place. As an example, volume concentration of macromolecules in the cytoplasm of Escherichia coli is as high as 30–40%, leading to significant deviations of macromolecular reaction rates and equilibria from those in diluted samples. The current consensus is that the functional outcomes of molecular crowding stem from two phenomena: hard-core repulsions, otherwise referred to as “entropic effects,” and soft chemical interactions. This year, we demonstrated that the entropic effects are generally more subtle than just the hard-core repulsions, because they necessarily include the phenomenon of forced macromolecule partitioning into protein cavities (Reference 1). With consequences for the selective sorting of large molecules in cells, studies of polymer partitioning into nanopores are also instructive for refining single-molecule sensing (Reference 2) as well as for polymer-assisted transport and packaging.
To address these questions, we analyzed forced size-dependent partitioning of binary mixtures of differently sized polyethylene glycols (PEGs) in nanosize pores. We probed three structurally different channels: VDAC from the outer mitochondrial membrane, bacterial porin OmpC (outer membrane protein C), and bacterial channel-forming toxin alpha-Hemolysin (Figure 1). Our interpretation was based on the idea that relatively less-penetrating polymers push the more easily penetrating ones into nanosize channels in excess of their bath concentration. All three channels exhibited forced partitioning that could be understood conceptually as well as quantitatively within a “polymers-pushing-polymers” model, allowing good estimates for the size-dependent pore penetration energy differences. As a result of our work, beyond proof of concept, forced partitioning in cells can now be recognized and used as a new tool in studies of molecule-specific transport and active osmotically regulated packaging. In particular, comparison of the theory with experiments has proven to be excellent for VDAC. Polymer partitioning data for the other two channels were consistent with theory if additional assumptions regarding the energy penalty of pore penetration are included. However, because of the several implicit assumptions, including those that idealized channel geometry, it is reasonable to expect deviations from the theoretical predictions. The obtained results demonstrate that the general concept of “polymers-pushing-polymers” is helpful in understanding and quantification of concrete examples of size-dependent forced partitioning of polymers into protein clefts and nanopores, with the obvious consequences for interpretation of molecular recognition under crowding conditions.
Progress in theoretical and experimental studies of the voltage-dependent anion channel of the outer mitochondrial membrane
Present understanding of molecular mechanisms of functioning of beta-barrel channels, such as VDAC, general and specific bacterial porins, and various toxin channels, is far behind the progress in the field of “conventional” highly selective ion channels. Specifically, mitochondrial channels constitute a separate class characterized by unique properties that distinguish them from other channels, including evolutionarily related bacterial porins. Mitochondrial channels are directly involved in regulation of the normal function of mitochondria and in metabolic changes in response to environmental challenges and different kinds of stress, such as apoptosis. For example, VDAC, the channel of the outer mitochondrial membrane, serves as a major pathway for metabolite exchange between the cytosol and mitochondria and thus controls a significant portion of outer-membrane permeability. Small ions and water-soluble mitochondrial metabolites, such as ATP and ADP, all cross the mitochondrial outer membrane through one pathway, the VDAC. Therefore, determination of the molecular basis of VDAC’s ion selectivity, metabolite permeability, and regulation is crucial to understanding its function and physiological role. Our recent progress in VDAC studies (Reference 3) demonstrates that the computational techniques not only complement experimental electrophysiological data but also lead to an a priori identification of binding sites for ATP or for cytosolic regulators of VDAC, thus connecting existing structures to the long-standing proposition that VDAC plays a crucial role in providing and regulating metabolite transport in and out of mitochondria. Using a very promising new computational technology based on the combination of 2-dimensional umbrella sampling simulations with the distributed replica-exchange protocol, we showed that ATP in the VDAC pore exhibits a surprisingly broad distribution of states. For the first time, our analysis accounted for the flexibility of ATP molecule, revealing 10 most probable structural clusters along the pore that define the structure and energetics of permeation pathways (Figure 2). Notably, one of the pathways can be associated with the highest possible ATP flux rates. It appears that, in a majority of cases, the rate-limiting step is dissociation of ATP from the alpha-helix of the VDAC N-terminus. Importantly, all basic residues in the VDAC’s N-terminus, which participate in formation of an ATP–binding site, were also found to bind to the acidic residues in the C-terminal tail of alpha-tubulin, a very efficient regulator of VDAC function. This conclusion is supported by our previous experiments and calculations, which show that ATP is excluded from the tubulin-blocked state of VDAC. Thus, the availability of the recently solved structures, our latest experimental data, and powerful state-of-the-art computational techniques, combined with modern analytical approaches, led to the breakthrough in our understanding of the relationship between VDAC function and structure.
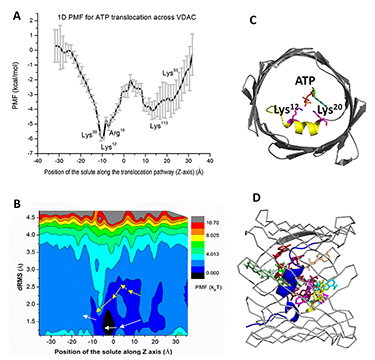
Click image to enlarge.
Figure 2. ATP translocation facilitated by interactions with VDAC pore residues (Reference 3)
One- (A) and two-dimensional (B) Potentials of Mean Force (PMF) for ATP transport across VDAC1 channel. In 2D PMF simulations, each replica (window) makes periodic exchange attempts with its four neighboring replicas. In each exchange cycle, exchange attempts are performed alternatively along the two dimensions to accelerate the travel through the extended ensemble. The permeation pathways mapped from simulations are shown in white arrows, while the pathway 5 from the Markov-State-Model (MSM) analysis is illustrated with yellow arrows. (C) The high-affinity site identified from 1D PMF computations. (D) The top 10 most likely ATP conformations along the permeation pathway are shown.
Physical theory of channel-facilitated transport
Physical theory of channel-facilitated transport gives us a set of rigorous tools for quantitative analysis of experimental findings. This year, we had extended our studies of entropic effects in transport problems. Whenever transport of particles takes place in the presence of three-dimensional non-uniform confining geometries, the description of the particle dynamics in terms of the one-dimensional Smoluchowski equation effectively introduces entropy potentials, which account for the variations in confinement geometry along a particle’s path. Such structures with varying confinement are ubiquitous in both technology and biology. Ion channels offer a class of probably the smallest structures in which the main concepts of confined diffusion are still applicable. One of our studies (Reference 4) addresses the range of validity of the reduction of axial diffusion in two-dimensional channels to the effective one-dimensional description in terms of the modified Fick-Jacobs equation. We demonstrated that such a reduction is applicable when the channel width variation rate does not exceed unity. In another study (Reference 5), we analyzed the diffusion of point particles in linearly corrugated two-dimensional channels. Such geometry allowed us to obtain an approximate analytical expression that gives the particle effective diffusivity as a function of the geometric parameters of the channel. To establish its accuracy and the range of applicability, we tested the expression against Brownian dynamics simulation results. The tests showed that the expression works very well for long channel corrugation periods, but fails when the period is not long enough compared with the minimum width of the channel. To address this deficiency, we proposed a simple empirical correction to the analytical expression: the “structure wavelength” correction. The resulting expression for the effective diffusivity proved to be in excellent agreement with the simulation results for all values of the channel corrugation period.
Publications
- Aksoyoglu MA, Podgornik R, Bezrukov SM, Gurnev PA, Muthukumar M, Parsegian VA. Size-dependent forced PEG partitioning into channels: VDAC, OmpC, and Alpha-Hemolysin. Proc Natl Acad Sci USA 2016;113:9003–9008.
- Kasianowicz JJ, Bezrukov SM. On “Three decades of nanopore sequencing”. Nat Biotech 2016;34:481-482.
- Noskov SY, Rostovtseva TK, Chamberlin AC, Teijido O, Jiang W, Bezrukov SM. Current state of theoretical and experimental studies of the voltage-dependent anion channel (VDAC). Biochim Biophys Acta 2016;1858:1778-1790.
- Berezhkovskii AM, Dagdug L, Bezrukov SM. Range of applicability of modified Fick-Jacobs equation in two dimensions. J Chem Phys 2015;143:164102.
- Verdel R, Dagdug L, Berezhkovskii AM, Bezrukov SM. Unbiased diffusion in two-dimensional channels with corrugated walls. J Chem Phys 2016;144:084106.
Collaborators
- Vicente M. Aguilella, PhD, Universidad Jaume I, Castellón, Spain
- Alphan Aksoyoglu, MS, University of Massachusetts Amherst, Amherst, MA
- Alexander M. Berezhkovskii, PhD, Division of Computational Bioscience, CIT, NIH, Bethesda, MD
- Susan K. Buchanan, PhD, Laboratory of Molecular Biology, NIDDK, Bethesda, MD
- Leonid V. Chernomordik, PhD, Section on Membrane Biology, NICHD, Bethesda, MD
- Leonardo Dagdug, PhD, Universidad Autónoma Metropolitana-Iztapalapa, Mexico City, Mexico
- David Hoogerheide, PhD, National Institute of Standards and Technology, Gaithersburg, MD
- Jennifer C. Lee, PhD, Biochemistry and Biophysics Center, NHLBI, Bethesda
- Ekaterina M. Nestorovich, PhD, The Catholic University of America, Washington, DC
- Sergei Y. Noskov, PhD, University of Calgary, Calgary, Canada
- Adrian Parsegian, PhD, University of Massachusetts Amherst, Amherst, MA
- Olga Protchenko, PhD, Liver Diseases Branch, NIDDK, Bethesda, MD
- Dan Sackett, PhD, Section on Cell Biophysics, NICHD, Bethesda, MD
- Gerhard Wagner, PhD, Harvard Medical School, Cambridge, MA
- Michael Weinrich, MD, Director, National Center for Rehabilitation Research, NICHD, Bethesda, MD
- David L. Worcester, PhD, National Institute of Standards and Technology, Gaithersburg, MD
- Joshua Zimmerberg, MD, PhD, Section on Cellular and Membrane Biophysics, NICHD, Bethesda, MD
Contact
For more information, email bezrukov@helix.nih.gov or visit http://smt.nichd.nih.gov.