Neuregulin–ErbB Signaling in Neuronal Development and Psychiatric Disorders

- Andres Buonanno, PhD, Head, Section on Molecular Neurobiology
- Detlef Vullhorst, PhD, Staff Scientist
- Irina Karavanova, PhD, Research Assistant
- Tanveer Ahmed, PhD, Postdoctoral Fellow
- Lalitha Kurada, PhD, Postdoctoral Fellow
- Miguel Skirzewski, PhD, Postdoctoral Fellow
- Larissa Erben, MS, Graduate Student
Failure of cortical microcircuits to properly regulate excitatory/inhibitory (EI) balance is a key feature in the etiology of several developmental psychiatric disorders and neurological diseases, such as schizophrenia, autism, ADHD, and epilepsy. EI balance is important to synchronize the firing pattern of local neuron ensembles, and its dysregulation can degrade cognitive functions and, in extreme cases, result in epileptiform activity. Network activity, in particular oscillatory activity in the gamma-frequency range (30–80 Hz), is altered in psychiatric disorders and may account for their cognitive and behavioral symptoms. We are interested in how Neuregulin and its receptor ErbB4, which are both genetically linked to psychiatric disorders, function in rodents in an activity-dependent fashion (i.e., experience) in the developing brain to regulate synaptic plasticity, neuronal network activity, and behaviors that model features of psychiatric disorders. We identified a functional interaction among Neuregulin/ErbB4, GABAergic, and dopamine signaling in GABAergic interneurons that is critical for understanding how Neuregulin can regulate EI balance and synchronous activity in neuronal networks, two processes that are important for cognitive functions altered in psychiatric disorders.
Our earlier studies demonstrated that, in the hippocampus and neocortex, expression of ErbB4, the major Neuregulin neuronal receptor, is restricted to GABAergic interneurons, particularly the parvalbumin-positive (Pv+) fast-spiking interneurons, which are critically important for modulating gamma oscillation induction and strength (i.e., power). Using genetically targeted mouse mutant models, we went on to show that Neuregulin–ErbB4 signaling regulates synaptic plasticity, neuronal network activity (i.e., gamma oscillations), and behaviors associated with psychiatric disorders. More recently, our group has been investigating other aspects of Neuregulin expression throughout the brain, its processing in response to neuronal activity, and its function in distinct neuronal populations of the developing and maturing nervous system. To achieve these goals, we are using a combination of techniques, including electrophysiological recordings in acute brain slices prepared from normal and genetically altered mice, multi-electrode field recordings from brains of freely moving rats, reverse-microdialysis neurochemistry, confocal fluorescence microscopy in fixed and live tissue, proteomics analyses, and behavioral testing. The ultimate goal of this multi-disciplinary approach is to generate holistic models to investigate the developmental impact of genes that modulate EI balance and neuronal network activity and that consequently affect behaviors and cognitive functions altered in psychiatric disorders.
Subcellular distribution of Neuregulin isoforms in central neurons
Numerous Neuregulins (NRGs) are generated by four different genes (NRG1–NRG4), distinct promoters (NRG1 types I, II and II), and alternative splicing, but the functional significance of this evolutionarily conserved diversity has, for over two decades, remained poorly understood. Our recent studies reveal that NRGs can be categorized by their distinct transmembrane topologies, which impart fundamentally different subcellular trafficking properties. As discussed in more detail in the sections below, NRGs whose pro-forms contain a single-pass transmembrane domain (TMD), such as NRG1 (types I and II) and NRG2, target the cell-body plasma membrane, where they accumulate at specialized contact sites with the underlying endoplasmic reticulum. Their ectodomains are cleaved by sheddases in an NMDA receptor activity–dependent manner and are then shed to signal in paracrine or autocrine fashion. Contrary to prior studies reporting NRG3 as a single-pass type I TMD protein, our analysis revealed that NRG3 is a dual-pass TMD protein. Interestingly, we found that, by contrast to the single-pass TMD NRGs, dual-pass NRG3 and NRG1 [type III; cysteine-rich domain (CRD)] are instead targeted to axons where they signal in juxtacrine mode (see Figure 1 for summary). The findings reveal a previously unknown functional relationship between membrane topology and subcellular targeting and suggest that single- and dual-pass TMD NRGs regulate neuronal functions in fundamentally different ways (Ahmed T, Vullhorst D et al. J Neurosci, in review). This work was supported by a Director's Investigator Award.
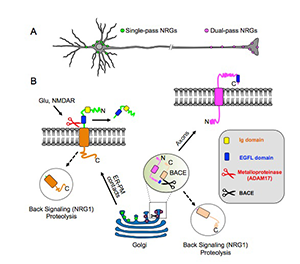
Click image to enlarge.
Figure 1. Transmembrane topology of unprocessed pro-NRGs determines their neuronal subcellular targeting and processing in central neurons.
A. Single-pass NRGs accumulate at subsurface cistern (SSC)–type ER–PM contact sites on the cell body and proximal dendrites to promote local NRG–ErbB signaling, whereas dual-pass NRGs accumulate on axons and axon terminals to drive long-range signaling. B. Single-pass NRGs are trafficked through the secretory pathway as unprocessed pro-forms and accumulate at SSC–type ER–PM contact sites. Ectodomain shedding in response to glutamate signaling via NMDA receptors occurs at the plasma membrane at the neuronal cell surface. By contrast, unprocessed dual-pass NRGs are retained in the Golgi, and intracellular BACE (beta-secretase) processing releases the amino-terminal proteolytic fragment and enables anterograde transport into axons. Both single- and dual-pass NRGs are processed by gamma-secretase to release carboxyl-terminal proteolytic fragments that can "back signal" to acutely regulate TRPV and alpha7-containing nACh receptors in axons (Hancock ML et al. J Cell Biol 2008;181:511; Canetta SE et al. PLoS One 2011;6:e2510) and gene expression in the nucleus (Bao J et al. J Cell Biol 2003;161:1133; Bao J et al. Nat Neurosci 2004;7:1250).
Unprocessed proNRG2 accumulates on top of subsurface cisterns, and its processing and shedding are regulated by NMDA receptor activity.
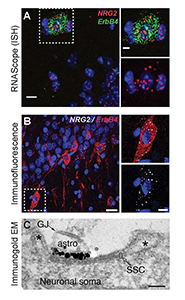
Click image to enlarge.
Figure 2. Neuregulin-2 is expressed in ErbB4–positive GABAergic interneurons and accumulates at SSCs.
A. Double fluorescence in situ hybridization (ISH) of NRG2/Gad67 and NRG2/ErbB4 in the mouse stratum oriens of CA1. B. NRG2 immunoreactivity in ErbB4+ interneurons in rat CA1 strata pyramidale and radiatum. C. Silver-enhanced immunogold EM of a DIV 35 hippocampal neuron with a patch of concentrated label for NRG2 at the plasma membrane atop intracellular membrane stacks characteristic of SSCs.
We found that proNRG2 localizes on top of subsurface cisterns (SSCs), a site of endoplasmic reticulum–plasma membrane junctions, where it accumulates at the center of doughnut-shaped Kv2.1 (a voltage-gated potassium channel) clusters at the edges of SSCs (Figure 2). Interestingly, treatment of neuronal cultures with glutamate or N-methyl-D-aspartate (NMDA) causes the rapid dispersal of both proteins from the SSC and processing of proNRG2 by sheddases to release the signaling-competent NRG2. We wished to determine the function of released NRG2 from GABAergic interneurons, which are known to express the ErbB4 receptor. In collaboration with Sanford Markey's group, we used ErbB4 immunoprecipitation from the soluble fraction of metabolically active synaptosomes followed by LC/MS/MS (liquid chromatography/tandem mass spectrometry) to characterize the ErbB4 proteome. Using this approach, we identified the GluN2B subunit of the NMDA receptor (NMDAR) as an ErB4–interacting protein. We confirmed the interaction in cultured hippocampal neurons, where NRG2 treatment was shown to enhance the internalization of GluN2B–containing, but not GluN2A–containing, NMDARs (Figure 3). Consistent with this observation, we found that NRG2 also caused a dramatic reduction of whole-cell NMDAR currents in dissociated hippocampal ErbB4–positive interneurons, but not in ErbB4–negative glutamatergic neurons. Lastly, using whole-cell voltage-clamp recordings in acute medial prefrontal cortical slices, we found that NRG2 selectively reduced NMDAR synaptic currents (EPSCs), but not AMPAR EPSCs, at glutamatergic synapses onto GABAergic interneurons. The results are consistent with the idea that the bidirectional signaling between NRG2/ErbB4 and NMDAR activity can play a major role in modulating the activity of GABAergic neurons and cortical E/I balance (Reference 1).
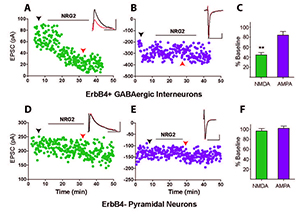
Click image to enlarge.
Figure 3. Neuregulin-2 selectively reduces NMDAR currents, but not AMPAR currents, in GABAergic interneurons.
A and B. Representative scatter plots of NMDAR– and AMPAR–mediated evoked EPSCs recorded from an ErbB4–positive GABAergic interneuron and (D and E) recorded from a pyramidal neuron. C and F. Summary graphs of NRG2 effects on NMDA and AMPA evoked excitatory postsynaptic currents (eEPSCs). Data normalized to baseline.
Neuregulin-2 ablation results in dopamine dysregulation and severe behavioral phenotypes relevant to psychiatric disorders.
While the neurophysiological and behavioral phenotypes of Nrg1–mutant mice have been investigated extensively, little is known about the function of NRG2 (see above), the closest NRG1 homolog. We found that NRG2 expression in the adult rodent brain does not fully overlap with NRG1 and is more extensive than originally reported, including expression in the striatum and medial prefrontal cortex (mPFC). We therefore generated Nrg2 knockout (KO) mice to study the homolog's function. Nrg2 KO mice have elevated extracellular dopamine levels in the dorsal striatum but reduced levels in the mPFC, similar to schizophrenia subjects. Nrg2 KOs also performed abnormally in a battery of behavioral tasks relevant to psychiatric disorders. The mutant mice exhibit hyperactivity in the open field, hypersensitivity to amphetamine, deficits in prepulse inhibition, reduced anxiety-like behavior in the elevated plus-maze, and antisocial behavior. Acute administration of clozapine (1mg/kg) increased the concentration of mPFC dopamine and improved performance in the T-maze alteration reward task (Figure 4). We also demonstrated that, in Nrg2 KOs, NMDA receptor synaptic currents are augmented at hippocampal glutamatergic synapses and more sensitive to ifenprodil, indicating an increased contribution of GluN2B–containing NMDARs (Yan et al. Mol Psychiatry, in review). Our findings reveal a novel role for NRG2 in the modulation of behaviors relevant to the core symptoms of schizophrenia.
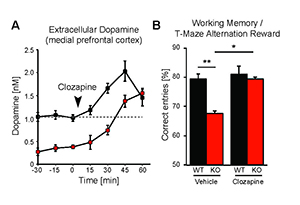
Click image to enlarge.
Figure 4. Administration of antipsychotic clozapine restores dopamine levels in the pre-frontal cortex of Neuregulin-2 knockout mice and improves performance on a cognitive task.
A. Extracellular dopamine levels in the mPFC of Nrg2 knockout (KO) mice and wild-type (WT) littermates before and after clozapine injection (arrowhead). B. Poorer performance by Nrg2 KO mice in a T-maze reward alternation task than by WT littermates (left) can be restored by clozapine treatment (right).
Analysis of ErbB4 function in mice harboring targeted mutations in GABAergic and dopaminergic neurons
Dysfunctional Neuregulin-ErbB4 signaling in the hippocampus, pre- frontal cortex (PFC), or striatum may contribute to alterations in dopamine (DA) function associated with several schizophrenia symptoms. Given that we showed NRG1 to acutely raise extracellular DA levels to regulate long-term potentiation (LTP) and gamma oscillations and that ErbB4 receptor expression is confined to GABAergic interneurons (cortex) and tyrosine hydroxylase positive (TH+) mesocortical DAergic neurons, we used genetic, biochemical, and behavioral approaches to measure DA function in the hippocampus, PFC, and striatum in mice harboring targeted mutations of ErbB4 in either PV+ or TH+ neurons. Interestingly, we found that, in contrast to GABAergic neurons, ErbB4 is highly expressed on the axons of DA neurons, suggesting that NRG/ErbB4 signaling may directly regulate the presynaptic function of these neurons. In collaboration with the laboratories of Susan Amara and Veronica Alvarez, we found that NRG regulates the increase in extracellular DA levels, at least in part, by DA transporter function. In contrast to mice harboring CNS–wide or GABAergic–restricted mutation of ErbB4, which show sensory-motor gating deficits and increases in motor activity, mice with ablation of ErbB4 in TH+ neurons only manifest behavioral deficits in cognitive-related tasks, such as performance on the T-maze, Y-maze, and Barnes maze (Skirzewski et al., in preparation). Our findings suggest that, in combination, direct effects of NRG/ErbB4 signaling in GABAergic and DAergic neurons regulate cortical circuits and DA homeostasis to affect numerous behaviors relevant to schizophrenia.
Effects of ketamine on cortical gamma oscillations and the role of dopamine receptors
Mounting evidence suggests that gamma oscillations are atypically high at baseline in disorders that affect attention, such as schizophrenia and ADHD. Ketamine, an antagonist of the NMDAR, elicits psychosis and affects cognitive functions in healthy individuals that phenocopy schizophrenia. In collaboration with the lab of Judith Walters, we are using multi-electrode recordings from the medial prefrontal cortex and dorsomedial thalamus of rats acutely treated with ketamine to analyze the effects of ketamine on gamma oscillations in vivo (Figure 5) and to determine whether and how D4 and ErbB4–targeting drugs can affect gamma oscillations in this rodent model with "face validity" for schizophrenia (Furth et al. Psychopharmacology, in review). We are also performing experiments to evaluate the effects of drugs targeting dopamine D4 receptors on attention and impulsivity behaviors in mice, using the five-choice serial-reaction time task (5CSRTT).
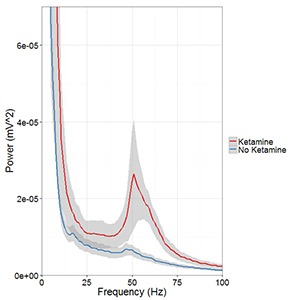
Click image to enlarge.
Figure 5. Ketamine raises gamma (40–65Hz) frequency power in the medial prefrontal cortex (mPFC) during controlled movement.
Multi-electrode recordings in the mPFC revealed an over three-fold increase in gamma (40–65Hz) frequency power while rats walked counter-clockwise at 9–10 revolutions per minute on a circular treadmill. Blue traces come from an epoch 30 minutes before ketamine administration and the red trace comes from epochs 15 minutes after ketamine administration.
Additional Funding
- Bench-to-Bedside Award (NHD15003-001-00001) "Selective Dopamine D4 Receptor-Targeting Compounds as Pro-Cognitive Drugs"
- NICHD DIR Director's Investigator Award (RRC# D-14-07). PI: Andres Buonanno; Co-PI: Juan Bonifacino. "Exploring the functional role of Neuregulin isoform diversity in CNS"
Publications
- Vullhorst D, Mitchell RM, Keating C, Roychowdhury S, Karavanova I, Tao-Cheng JH, Buonanno A. A negative feedback loop controls NMDA receptor function in cortical interneurons via neuregulin 2/ErbB4 signalling. Nat Commun 2015;6:7222.
- Canfield K, Li J, Wilkins OM, Morrison MM, Ung M, Wells W, Williams CR, Liby KT, Vullhorst D, Buonanno A, Hu H, Schiff R, Cook RS, Kurokawa M. Receptor tyrosine kinase ERBB4 mediates acquired resistance to ERBB2 inhibitors in breast cancer cells. Cell Cycle 2015;14:648-655.
Collaborators
- Veronica Alvarez, PhD, Section on Neuronal Structure, NIAAA, Rockville, MD
- Susan G. Amara, PhD, Laboratory of Molecular and Cellular Neurobiology, NIMH, Bethesda, MD
- Jung Hwa (Susan) Cheng, PhD, EM Facility, NINDS, Bethesda, MD
- Jennie Garcia-Olivares, PhD, Laboratory of Molecular and Cellular Neurobiology, NIMH, Bethesda, MD
- Idit Golani, MD, Technion–Israel Institute of Technology, Haifa, Israel
- Ilana Kremer, MD, Technion–Israel Institute of Technology, Haifa, Israel
- Sanford P. Markey, PhD, Laboratory of Neurotoxicology, NIMH, Bethesda, MD
- Alon Shamir, PhD, Mazra Mental Health Center, Akko, Israel
- Jung-Hoon Shin, PhD, Section on Neuronal Structure, NIAAA, Rockville, MD
- Judith R. Walters, PhD, Experimental Therapeutics Branch, NINDS, Bethesda, MD
Contact
For more information, email buonanno@mail.nih.gov or visit http://smn.nichd.nih.gov.