Nervous System Development and Plasticity
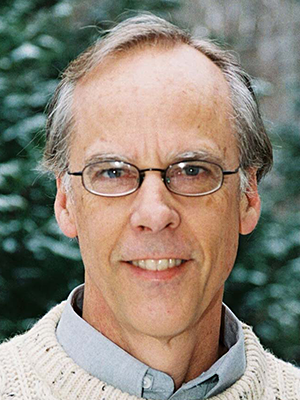
- R. Douglas Fields, PhD, Head, Section on Nervous System Development and Plasticity
- Philip Lee, PhD, Staff Scientist
- Dipankar Dutta, PhD, Visiting Fellow (Henry Jackson Foundation)
- Dong Ho Woo, PhD, Visiting Fellow
- William Huffman, MA, Technician
Healthy brain and cognitive development in children is central to the mission of NICHD. Unlike the brains of most animals, the human brain continues to develop postnatally, through adolescence and into early adulthood. The prolonged postnatal period of brain development allows environmental experiences to influence brain structure and function, rather than having brain function specified entirely by genes. Activity-dependent plasticity also compensates for developmental defects and brain injury. Our research is concerned with understanding the molecular and cellular mechanisms by which functional activity in the brain regulates development of the nervous system during late stages of fetal development and early postnatal life. We are especially interested in novel mechanisms of activity-dependent nervous system plasticity that are particularly relevant to the period of childhood and of those that operate beyond the synapse and beyond the neuron doctrine. Our work has three main areas of emphasis: myelination and neuron-glia interactions, cellular mechanisms of learning, and gene regulation by neuronal firing.
Traditionally, the field of activity-dependent nervous system development has focused on synapses, and we continue to explore synaptic plasticity. However, our research is also advancing understanding of how non-neuronal brain cells (glia) sense neural impulse activity and how activity-dependent regulation of glia contributes to development, plasticity, and the cellular mechanisms of learning. A major emphasis of our current research is to understand how myelin (white matter in the brain) is regulated by functional activity. By changing conduction velocity, activity-dependent myelination may be a non-synaptic form of plasticity, regulating nervous system function by optimizing the speed and synchrony of information transmission through neural networks. Our studies identified several cellular and molecular mechanisms for activity-dependent myelination, and the findings have important implications for normal brain development, learning and cognition, and psychiatric disorders. Our research showing that myelination of axons by glia (oligodendrocytes and Schwann cells) is regulated by impulse activity provides evidence for a new form of nervous system plasticity and learning that would be particularly important in child development, given that myelination proceeds throughout childhood and adolescence. The mechanisms we identified suggest that environmental experience may alter myelin formation in an activity-dependent manner, thereby improving function based on experience.
Learning is perhaps the most important function of childhood. Our research is delineating the molecular mechanisms that convert short-term memory into long-term memory. While we continue our long-standing research on synaptic plasticity, our laboratory is actively exploring new mechanisms of nervous system plasticity during learning that extend beyond the neuron doctrine, such as neurons firing antidromically (backwards) and the release of neurotransmitters along axons. We are investigating how gene expression necessary for long-term memory is controlled and how intrinsic activity in the brain (oscillations and neuronal firing) forms memories. Our recent research showed that neurons in the hippocampus fire antidromically during sharp-wave ripple complexes, which are most frequent during slow-wave sleep, and that the firing reduces the strength of all synapses on that neuron (action potential–induced long-term depression or AP-LTD).
Information in the nervous system is encoded in the temporal pattern of action potential firing. If functional experiences produce lasting effects on brain development and plasticity, specific genes must be regulated by specific patterns of impulse firing. We verified the hypothesis and are determining how different patterns of neural impulses regulate specific genes controlling development and plasticity of the nervous system and how impulse activity affects neurons and glia.
Regulation of myelination by neural impulse activity
Myelin, the multilayered membrane of insulation wrapped around nerve fibers (axons) by glial cells (oligodendrocytes), is essential for nervous system function, increasing conduction velocity at least 50-fold. Myelination is an essential part of brain development, but the processes controlling myelination of appropriate axons are not well understood. Myelination begins in late fetal life and continues throughout childhood and adolescence, but myelination of some brain regions is not complete until an individual's early twenties. Our research shows that neurotransmitters that are released along axons firing action potentials activate receptors on myelinating glia (Schwann cells in the peripheral nervous system and oligodendrocytes in the central nervous system) as well as astrocytes and other cells, which in turn release growth factors or cytokines that regulate development of myelinating glia.
Induction of myelination by action potentials
In addition to establishing the effects of impulse activity on proliferation and development of myelinating glia, we determined that release of the neurotransmitter glutamate from vesicles along axons promotes the initial events in myelin induction, including stimulating the formation of cholesterol-rich signaling domains between oligodendrocytes and axons and increasing the local synthesis of myelin basic protein, the major protein in the myelin sheath, through Fyn kinase–dependent signaling. Axon-oligodendrocyte signaling would thus promote myelination of electrically active axons to regulate neural development and neural function according to environmental experience. The findings are also relevant to such demyelinating disorders as multiple sclerosis and to remyelination after axon injury.
Synaptic and nonsynaptic transmission in myelination
The surprising discovery of synapses formed on glial progenitors—oligodendrocyte progenitor cells (OPCs, also called NG2 cells)—has remained enigmatic for over a decade. The cells mature to form myelin insulation on axons, and a leading hypothesis is that these synapses may stimulate myelination selectively on electrically active axons, which would have significant effects on neural function, given that myelin increases the speed of impulse transmission. Using an in vitro system, we found that oligodendrocytes preferentially myelinate electrically active axons but that synapses from axons onto myelin-forming oligodendroglial cells are not required. Instead, vesicular release at non-synaptic axo-glial junctions induces myelination. Axon release of neurotransmitter from vesicles that accumulate in axon varicosities induces a local rise in cytoplasmic calcium in glial cell processes at such nonsynaptic functional junctions, and this signaling stimulates local translation of myelin basic protein to initiate myelination. As mentioned above, preferential myelin induction on electrically active axons would have profound effects on circuit function by increasing conduction velocity and would thus provide another mechanism of plasticity complementing synaptic plasticity. These new findings may also have implications for disease, including psychiatric illness and impaired remyelination after conduction block in multiple sclerosis and other demyelinating disorders.
Myelin in activity-dependent plasticity
Although the significance of myelin has been traditionally viewed in terms of conduction failure and spike-time arrival in determining synaptic function and plasticity, we are exploring how myelination affects the frequency, phase, and amplitude coupling of oscillations in the brain, as well as the propagation of brain waves. Abnormalities in brain waves and synchrony are associated with many psychiatric and developmental conditions, including, among others, schizophrenia, epilepsy, dyslexia, and autism.
Gulf War Illness
After decades of research there is still no understanding of how a large group of Gulf War veterans became chronically ill with Gulf War Illness. It is believed that exposure to low levels of sarin nerve gas and combinations of organophosphate insecticides, which impair synaptic function, may be responsible. Our recent discovery that glutamatergic transmission between axons and oligodendrocytes triggers myelination led us to propose that impairments in myelination due to disrupted neurotransmission from axons to oligodendrocytes may be an underlying cause of Gulf War Illness. Funded in part by a grant from the Department of Defense, we are investigating this hypothesis as part of an international consortium of researchers studying Gulf War Illness, headed by Kimberly Sullivan.
Myelin damage in children exposed to pesticides
Children are uniquely vulnerable to uptake of pesticides, which act chemically through the same mechanism as nerve gas. Exposure, through ingestion and inhalation, is higher than for adults because of their greater intake of food and fluids per pound of body weight. In 2003, FDA monitoring determined that 49% of fruit, 29% of vegetables, and 26% of grain products produced in the United States have pesticide residue. The concentrations of pesticides in imported fruits and vegetables are higher, and 5–7% of imported foods with pesticide residue have levels of contamination above the legal tolerance limit for consumption. Fetal development is compromised by parental and maternal exposure to organophosphate pesticides. The neuro-developmental effects are well documented; they include developmental delay, lower IQ, and ADHD. Our research is thus exploring the possible involvement of myelin damage in the adverse developmental effects of pesticides on children.
Synaptic plasticity
It is widely appreciated that there are two types of memory: short-term and long-term, and that sleep has a critical role in memory consolidation. Gene expression is necessary to convert short-term into long-term memory, but it is not known how signals reach the nucleus to initiate this process or what genes control strengthening and weakening of synapses in association with learning. Long-term potentiation (LTP) and long-term depression (LTD) are two widely studied forms of synaptic plasticity that can be recorded electrophysiologically in the hippocampus and are believed to represent a cellular basis for memory. We use electrophysiology and cDNA microarrays to investigate the signaling pathways, genes, and proteins involved in LTP and LTD. The work is contributing to a better understanding of how regulatory networks are controlled by the appropriate patterns of impulses, leading to different forms of synaptic plasticity, and is identifying new molecular mechanisms regulating synaptic strength.
Homeostatic controls are required to prevent a neural network from becoming incapacitated, and sleep is thought to be an important period for LTD and dendritic remodeling in memory consolidation. Although most studies of learning and memory use sensory stimulation, intrinsic activity in the brain, in particular oscillatory firing at different characteristic frequencies, is critical in information processing and learning. The brain's intrinsic activity includes many non-traditional modes of neuronal firing. In the hippocampus high-frequency oscillations affect synaptic plasticity in the process of memory consolidation during slow-wave sleep and periods of quiet wakefulness During these periods, neural sequences learned while encoding sensory information are replayed, and CA1 neurons in the hippocampus fire antidromically in brief high-frequency oscillations called sharp-wave ripple complexes. Action potential firing during sharp-wave ripple complexes is initiated in the distal axon and propagates back into the cell body and dendrites. Our studies show that such antidromic firing reduces the strength of all synaptic inputs to the neuron (AP–LTD) and that the synapses then become sensitized to strengthening by subsequent sensory input. The process of globally reducing synaptic strength participates in the formation of transiently stable functional assemblies of neurons and is necessary for incorporating new information together with existing memories to form a schema, or coherent memory, combining multiple sensations and temporal sequence into a cognitive framework.
The neurotrophin, brain-derived neurotrophic factor (BDNF) has been implicated in many forms of synaptic plasticity, nervous system development, and nervous system disorders. Our research shows that a rapid and persistent down-regulation of different mRNA transcripts of the BDNF gene accompanies AP-LTD. Moreover, AP-LTD is abolished in mice with the Bdnf gene knocked out in CA1 hippocampal neurons. Homeostatic controls are required to prevent a neural network from becoming incapacitated, and sleep is thought to be an important period for LTD and dendritic remodeling in memory consolidation. Given the well established role of this neurotrophin in increasing synaptic strength, promoting synaptogenesis and dendritic complexity, the rapid reduction of BDNF mRNA during AP-LTD could contribute to reducing synaptic strength during AP-LTD and promote synaptic remodeling.
Regulation of gene expression by action-potential firing patterns
To determine how gene expression in neurons and glia is regulated by impulse firing, we stimulate nerve cells to fire impulses in differing patterns by delivering electrical stimulation through platinum electrodes in specially designed cell culture dishes. After stimulation, we measured mRNA and protein expression by gene arrays, quantitative RT-PCR (reverse transcriptase–polymerase chain reaction), RNAseq, microarray, Western blot, and immunocytochemistry. The results confirm our hypothesis that precise patterns of impulse activity can increase or reduce expression of specific genes (in neurons and glia). The experiments are revealing signaling and gene-regulatory networks that respond selectively to appropriate temporal patterns of action potential firing. Temporal aspects of intracellular calcium signaling are particularly important in regulating gene expression according to neural impulse firing patterns in normal and pathological conditions. Our findings thus provide a deeper understanding of how nervous system development and plasticity are regulated by information coded in the temporal pattern of impulse firing in the brain. The findings are also relevant to chronic pain as well as to the regulation of nervous system development and myelination by functional activity.
In collaboration with David Clark, we are investigating chromatin structure and remodeling in neurons and glia. This research is supported in part by a NICHD DIR Director’s award jointly to Drs. Clark and Fields.
Additional Funding
- DOD Grant: 2012, Gulf War Illness Research Program Consortium Award, CDMRP Number: GW120037, Project Duration: 48 months
- NICHD DIR Director’s Award
Publications
- Wake H, Ortiz FC, Woo DH, Lee PR, Angulo MC, Fields RD. Nonsynaptic junctions on myelinating glia promote preferential myelination of electrically active axons. Nat Commun 2015;6:7844.
- Fields RD. A new mechanism of nervous system plasticity: activity-dependent myelination. Nat Rev Neurosci 2015;16(12):756-767.
- Filley CM, Fields RD. White matter and cognition: making the connection. J Neurophysiol 2016;116:2093-2104..
- Bukalo O, Lee PR, Fields RD. BDNF mRNA abundance regulated by antidromic action potentials and AP-LTD in hippocampus. Neurosci Lett 2016;635:97–102.
- Ravin R, Blank PS, Busse B, Ravin N, Vira S, Bezrukov L, Waters H, Guerrero-Cazares H, Quinones-Hinojosa A, Lee PR, Fields RD, Bezrukov SM, Zimmerberg J. Blast shockwaves propagate Ca(2+) activity via purinergic astrocyte networks in human central nervous system cells. Sci Rep 2016;6:25713.
Collaborators
- Maria Cecilia Angulo, PhD, INSERM, Université Paris Descartes, Paris, France
- Peter J. Basser, PhD, Section on Quantitative Imaging and Tissue Sciences, NICHD, Bethesda, MD
- David Clark, PhD, Section on Chromatin and Gene Expression, NICHD, Bethesda, MD
- Fernando Ortiz, PhD, Université René Descartes, Paris, France
- Rea Raven, PhD, Intramural Research Programs, NIBIB, Bethesda, MD
- Pajevic Sinisa, PhD, Division of Computational Bioscience, Center for Information Technology, NIH, Bethesda, MD
- Kimberly Sullivan, PhD, Boston University School of Public Health, Boston, MA
- Hiroaki Wake, PhD, National Institute for Basic Biology, Okazaki, Japan
- Joshua Zimmerberg, MD, PhD, Section on Cellular and Membrane Biophysics, NICHD, Bethesda, MD
Contact
For more information, email fieldsd@mail.nih.gov or visit http://nsdps.nichd.nih.gov.