Evolving Mechanisms of Transcriptional Control in Mammals
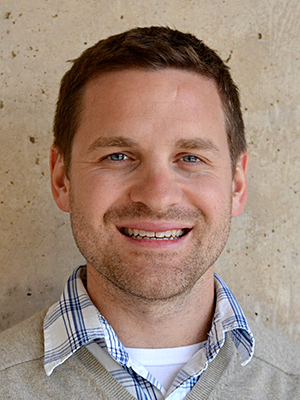
- Todd S. Macfarlan, PhD, Head, Unit on Mammalian Epigenome Reprogramming
- Carson Miller, PhD, Postdoctoral Fellow
- Gernot Wolf, PhD, Visiting Fellow
- Peng Yang, PhD, Visiting Fellow
- Sherry Ralls, BA, Biologist
- Don Hoang, BS, Postbaccalaureate Fellow
- Angela Maggio, BS, Postbaccalaureate Fellow
- Matthew Tinkham, BS, Postbaccalaureate Fellow
- Justin Demmerle, BA, Graduate Student
- Yixuan Wang, PhD, Collaborator
The “epigenome” refers to the heritable chemical changes in DNA and histone proteins that can be passed on through cell division and potentially across generations. Dramatic reprogramming of the epigenome begins at conception and continues throughout embryonic and postnatal development, underlying the gene-expression changes that drive the generation of diverse cell types, which make up complex multicellular organisms. By exploring transcription factors that recognize parasitic mobile DNA elements to initiate stable heterochromatic silencing, we study how the epigenetic state of mammals is initially established in early development. We also study how one large family of these parasitic elements, the endogenous retroviruses or ERVs, play an important role in development and evolution of new traits in mammals by rewiring gene expression networks. We also explore how the epigenetic state of a cell works in conjunction with combinatorial codes of transcription factors to generate the huge diversity of cell types required for mammalian development.
Retroviruses pose a threat to human health by infecting somatic cells, but retroviruses have also been infecting our mammalian ancestors for millions of years, accumulating in the germ-line as ERVs, which account for about 10% of our genomic DNA. My laboratory studies ERVs from two perspectives, as (1) as parasites that must be kept in check by the host to prevent widespread viral activation and (2) symbionts that can be co-opted by the host for evolutionary advantage. Our long-term objective is to understand how the host has adapted recognition machinery to establish epigenetic silencing of ERVs, how ERVs sometimes evade these silencing mechanisms, and how this “arms race” between ERVs and the host have led to co-option of viral regulatory sequences that likely contributed to the evolution of mammals. We hypothesize that the rapidly diversifying Krüppel-associated box–zinc finger protein (KRAB-ZFP) family of transcription factors plays a critical role in the recognition and silencing of ERVs and nearby genes, and we are taking systematic genetic and biochemical approaches to explore this function.
KRAB-ZFPs have emerged as candidates that recognize ERVs. KRAB-ZFPs are rapidly evolving transcriptional repressors that emerged in tetrapods. They make up the largest family of transcription factors in mammals (estimated to be about 200–300 in mice and humans)1. Each species has its own unique repertoire of KRAB-ZFPs, with a small number shared with closely related species and a larger fraction specific to each species. Despite their abundance, little is known about their physiological functions. KRAB-ZFPs consist of an N-terminal KRAB domain that binds to the co-repressor KAP12 and a variable number of C-terminal C2H2 zinc finger domains that mediate sequence-specific DNA binding. KAP1 directly interacts with the KRAB domain3, which recruits the histone methyltransferase SETDB1 and heterochromatin protein 1 (HP1) to initiate heterochromatic silencing4,5. Several lines of evidence point to a role for the KRAB-ZFP family in ERV silencing. First, the number of C2H2 zinc finger genes in mammals correlates with the number of ERVs6. Second, the KRAB-ZFP protein ZFP809 was isolated based on its ability to bind to the primer binding site for proline tRNA (PBSPro) of murine leukemia virus (MuLV)7. Third, deletion of the KRAB-ZFP co-repressors Trim28 or Setdb1 leads to activation of many ERVs8,9. We have thus begun a systematic interrogation of KRAB-ZFP function as a potential adaptive repression system against ERVs.

Click image to enlarge.
ZFP809 represses VL30Pro LTR (long-term repeat) retroelements.
a. Model of KRAB-ZFP protein function. KRAB-ZFPs consist of KRAB–repression domain that interacts with KAP1 to assemble a corepressor complex, establishing stable epigenetic silencing of targets. Tandem C2H2 zinc finger (ZF) domains mediate sequence-specific DNA interactions, with each ZF interacting with three nucleotides of target DNA. b. Heat map plots of ChIP-seq data (with indicated antibodies) of Flag-ZFP809 peak regions. Peaks were centered on PBSPro–like motif and grouped according to repeat masker annotation. c. RNA-seq differential expression analysis of all repeat masker annotated elements in Zfp809 wild-type (WT) vs. knockout (KO) spleen. Red dots indicate VL30Pro elements, most of which are reactivated in KOs.
We focused on ZFP809 as a likely ERV–suppressing KRAB-ZFP given that it was originally identified as part of a repression complex that recognizes infectious MuLV via direct binding to the 18 nt Primer Binding Site for Proline (PBSPro) sequence7,10. We hypothesized that ZFP809 might function in vivo to repress other ERVs that utilized the PBSPro. Using ChIP-seq of epitope-tagged ZFP809 in embryonic stem cells (ESCs) and embryonic carcinoma (EC) cells, we determined that ZFP809 bound to several sub-classes of ERV elements via the PBSPro. We generated Zfp809 knockout mice to determine whether ZFP809 was required for VL30Pro (VL30 is an ERV) silencing. We found that Zfp809 knockout tissues displayed high levels of VL30Pro elements and that the targeted elements display an epigenetic shift from repressive epigenetic marks (H3K9me3 and CpG methylation) to active marks (H3K9Ac and CpG hypo-methylation). ZFP809–mediated repression extended to a handful of genes that contained adjacent VL30Pro integrations. Furthermore, using a combination of conditional alleles and rescue experiments, we determined that ZFP809 activity was required in development to initiate silencing, but not in somatic cells to maintain silencing. The studies provided the first demonstration for the in vivo requirement of a KRAB-ZFP in the recognition and silencing of ERVs.
As a follow-up to our studies on ZFP809, we began a systematic analysis of KRAB-ZFPs using a medium-throughput ChIP-seq screen and functional genomics of KRAB-ZFP clusters and individual KRAB-ZFP genes. Our ChIP-seq data demonstrate that the majority of recently evolved KRAB-ZFP genes interact with and repress distinct and partially overlapping ERV targets. This is supported by a recent knockout mouse line lacking around 17 KRAB-ZFPs (generated with CRISPR/Cas9 engineering) that displays an ERV reactivation phenotype. Although our data show that many KRAB-ZFPs repress ERVs, we also found that more ancient KRAB-ZFPs that emerged in a human/mouse common ancestor do not bind to and repress ERVs. One of these KRAB-ZFPs plays an important role in silencing a key developmental gene that may have played a critical role in the onset of viviparity in mammals.
1Urrutia R. KRAB-containing zinc-finger repressor proteins. Genome Biol 2003;4:231.
2Friedman JR et al. KAP-1, a novel corepressor for the highly conserved KRAB repression domain. Genes Dev 1996;10:2067-2078.
3Peng H et al. Biochemical analysis of the Kruppel-associated box (KRAB) transcriptional repression domain. J Biol Chem 2000;275:18000-18010.
4Ryan RF et al. KAP-1 corepressor protein interacts and colocalizes with heterochromatic and euchromatic HP1 proteins: a potential role for Kruppel-associated box-zinc finger proteins in heterochromatin-mediated gene silencing. Mol Cell Biol 1999;19:4366-4378.
5Schultz DC, Ayyanathan K, Negorev D, Maul GG, Rauscher FJ 3rd. SETDB1: a novel KAP-1-associated histone H3, lysine 9-specific methyltransferase that contributes to HP1-mediated silencing of euchromatic genes by KRAB zinc-finger proteins. Genes Dev 2002;16:919-932.
6Thomas J H, Schneider S. Coevolution of retroelements and tandem zinc finger genes. Genome Res 2011;21:1800-1812.
7Wolf D, Goff SP. Embryonic stem cells use ZFP809 to silence retroviral DNAs. Nature 2009;458:1201-1204.
8Rowe HM et al. KAP1 controls endogenous retroviruses in embryonic stem cells. Nature 2010;463:237-240.
9Matsui T et al. Proviral silencing in embryonic stem cells requires the histone methyltransferase ESET. Nature 2010;464:927-931.
10Wolf D, Goff SP. TRIM28 mediates primer binding site-targeted silencing of murine leukemia virus in embryonic cells. Cell 2007;131:46-57.
Additional Funding
- Human Placenta Project ($30K): “Quantifying placental gene expression from maternal plasma-isolated cell-free nucleic acids for real-time monitoring of placental health”
Publications
- Wolf G, Greenberg D, Macfarlan TS. Spotting the enemy within: targeted silencing of foreign DNA in mammalian genomes by the Kruppel-associated box zinc finger protein family. Mobile DNA 2015;6:17.
- Wolf G, Macfarlan TS. Revealing the complexity of retroviral repression. Cell 2015;163:30-32.
- Geeven G, Zhu Y, Kim BJ, Bartholdy BA, Yang SM, Macfarlan TS, Gifford WD, Pfaff SL, Verstegen MJ, Pinto H, Vermunt MW, Creyghton MP, Wijchers PJ, Stamatoyannopoulos JA, Skoultchi AI, de Laat W. Local compartment changes and regulatory landscape alterations in histone H1-depleted cells. Genome Biol 2015;289.
- Wasson JA, Simon AK, Myrick DA, Wolf G, Driscoll S, Pfaff SL, Macfarlan TS, and Katz DJ. Maternally provided LSD1/KDM1A enables the maternal-to-zygotic transition and prevents defects that manifest postnatally. eLife 2016;e08848.
- Thompson PJ, Macfarlan TS, Lorincz MC. Long terminal repeats: from parasitic elements to building blocks of the transcriptional regulatory repertoire. Mol Cell 2016;163:30-32.
- Wang C, Lee J-E, Lai B, Macfarlan TS, Xu B, Zhuang L, Liu C, Peng W, Ge K. Enhancer priming by H4K4 methyltransferase MLL4 controls cell fate transition. Proc Natl Acad Sci USA 2016;113(42):11871-11876.
Collaborators
- Kai Ge, PhD, Adipocyte Biology and Gene Regulation Section, NIDDK, Bethesda, MD
- David J. Katz, PhD, Emory University School of Medicine, Atlanta, GA
- Keiko Ozato, PhD, Section on Molecular Genetics of Immunity, NICHD, Bethesda, MD
Contact
For more information, email todd.macfarlan@nih.gov or visit http://macfarlan.nichd.nih.gov.