Global Regulation of Gene Expression by ppGpp
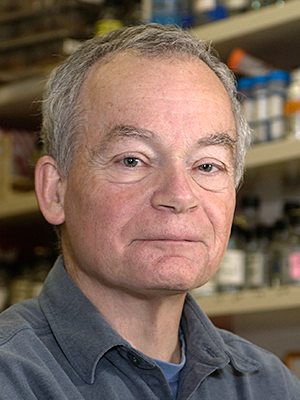
- Michael Cashel, MD, PhD, Head, Section on Molecular Regulation
- Llorenc Fernandez-Coll, PhD, Postdoctoral Intramural Research Training Award Fellow
- Suanam Vij, AB, Postbaccalaureate Intramural Research Training Award Fellow
Our research is aimed at a fundamental molecular understanding of the regulatory connections between stress and bacterial growth (in E. coli, for example) that are mediated by two signal nucleotide analogs, pppGpp and ppGpp, abbreviated as (p)ppGpp. The analogs differ from GTP and GDP simply by the presence of a pyrophosphate esterified on the 3′-ribose hydroxyl of GTP or GDP. When these analogs were discovered nearly 50 years ago, they represented a new structural family of nucleotide second messengers, the first being the cyclic purines c-AMP and c-GMP. Additional cyclic dinucleotides (c-diGMP, c-diAMP, and c-GAMP) are found in prokaryotes and eukaryotes. Together, these comprise extensive nucleotide-centric regulatory networks that function to sense damaged DNA or RNA, leading to protecting hosts from foreign invasions of nucleic acids (Burroughs AM et al. Nucleic Acids Res 2015;43:1063; Burroughs AM, Aravind L. Nucleic Acids Res 2016;44:8525). In contrast, (p)ppGpp seems to have arisen to address more primordial functions, namely protecting bacterial cells from nutritional and physical sources of stress that impair growth.
The importance of ubiquitous (p)ppGpp in bacteria is that accumulation of these compounds is triggered by exquisitely sensitive sensing of even minor disturbances in fundamental metabolism of amino acids, carbohydrates, or lipids. The ensuing response is often called the stringent response, which operates incrementally over about a 100–fold range of elevated (p)ppGpp concentrations, to reach a maximum equivalence with GTP levels. As a first approximation, the full-blown stringent response dramatically slows bacterial growth and renders cells semi-dormant. One common physiological effect is to limit synthesis of the translational apparatus. The benefit of this adjustment can be thought of as conserving resources not needed when growth slows or stops. The change is accompanied by coordinating many other stress-specific adjustments of global gene expression to form elements that minimize damage and ultimately adapt to overcome the stress.
The fundamental understanding of (p)ppGpp regulation has emerged to be important for clinical microbiology. Almost all bacterial pathogens survive in cellular hosts because they can overcome the stress conditions occasioned by intracellular host defenses. The stringent response is triggered by many such stresses. Together with the apparent absence of (p)ppGpp in animal cells, this has given rise to efforts to screen for novel chemical agents that eliminate (p)ppGpp, thereby immobilizing bacterial adaptation to stress and compromising pathogenicity. Anti-biofilm D-amino acid–containing peptides with amphipathic helices can block (p)ppGpp accumulation (Pletzer D et al. Curr Opin Microbiol 2016;33:35). Some of these peptides have emerged as effective therapy for model subcutaneous abscesses caused by methicillin-resistant (MRSA) Staphylococcus aureus (de la Fuente-Núñez C et al. PLoS Pathog 2014;10:e1004152). More generally, pharmacological targets of almost all antibiotics are typically inhibitors of essential metabolic pathways that are robust in growing cells. Given that (p)ppGpp occasions a semi-dormant state, as a first approximation, this is taken to explain how very rare instances of random cells with spontaneous high (p)ppGpp in a growing population of sensitive cells become antibiotic tolerant (Hauryliuk V et al. Nat Rev Microbiol 2015;13:298), leading to the persistence of the tolerant pathogens during antibiotic treatment, which resume growth and host damage when the antibiotic is removed. The phenomenon of pathogen persistence is probably related to carrier states of pathogens that persist even in the absence of antibiotics, although the mechanism is less clear. For example, in M. tuberculosis, the frequencies of the carrier state in animal models can be shown to be reduced in the absence of (p)ppGpp (Primm TP et al. J Bacteriol 2000;182:4889). Our research last year involved pGpp and (p)ppApp, described in earlier reports as a potentially new member of the noncyclic (p)ppGpp family. Progress on the (p)ppApp work has come through collaboration with a former lab member, Katarzyna Potrykus. This year, we collaborated in uncovering a facilitating role for ppGpp in the process of transcription-coupled repair of DNA damage.
(p)ppApp
Initial studies that led to a focus on (p)ppApp were presented in earlier reports. The studies were prompted by the existence of small single-domain hydrolases, called MESH (Metazoan E. coli Spot Homologs), shown by another laboratory. The enzymes are single-domain hydrolases with structural homology to known (p)ppGpp hydrolase domains present in bifunctional synthetases. As mentioned, all (p)ppGpp hydrolases remove a pyrophosphate residue and also are dependent on Mn++. A pivotal discovery made by Katarzyna Potrykus was that the MESH hydrolases display a degenerate substrate specificity in that they cleave (p)ppApp as well as (p)ppGpp. A concerted effort of ours, reported earlier, was to exploit the known crystal structures of MESH hydrolases with (p)ppGpp–specific hydrolase in order to derive a protein that could hydrolyze (p)ppApp but not (p)ppGpp. This was not, however, successful. Potrykus identified bacterial genomes that encoded MESH homologs. Several of these proteins were purified and, indeed, one was found that actively hydrolyzes (p)ppApp but not (p)ppGpp. The characterization of the enzymes used new NAD(P)–coupled spectrophotometric assays to measure hydrolysis rates of the nucleotides. The assays were devised here and developed further by Nathan Thomas, a postbaccalaureate fellow, whose work won a first prize NICHD poster award last year. The existence of the (p)ppApp–specific enzyme now permits one to screen for the cellular targets of the hydrolase. Such targets should validate (p)ppApp as an addition to the (p)ppGpp family of nucleotides. Many potential targets exist, given that they include any adenylate derivative with a free 3′-ribose hydroxyl; examples include Coenzyme A, FAD, and NAD, all known to be synthesized in vitro by a pyrophosphoryl transferase from a species of Streptomyces.
ppGpp facilitates transcription-dependent DNA repair.
Earlier reports from other labs suggested that maintenance of genomic integrity falls within the realm of (p)ppGpp–centric regulation. We collaborated in a study that further defined one aspect of these effects. The first clue was finding that high cellular basal levels of ppGpp conferred resistance to UV damage or to genotoxic bulky DNA adducts, when compared with ppGpp–deficient (ppGpp0) cells. Similar exposures of wild-type cells with low basal levels resulted in a transient ppGpp accumulation with levels increased far above basal values by 15 min, which thereafter decayed with a half-life of about 10 min. Evidence that repair of the DNA damage is directly linked to transcription came from the observation that repair of thymine dimers formed by UV irradiation occurred predominantly on the transcribed strand. The Mfd protein is known to participate in transcription-coupled repair through binding to the leading surface of RNA polymerase (RNAP). The binding event is thought to position the stalled enzyme near bulky DNA lesion, which allows recruitment of the proteins that initiate nucleotide excision repair. Subsequent binding of the UvrD helicase allows the RNAP to back up on the template to expose the DNA damaged region. The “backtracking” allows entry of additional nucleotide excision repair enzymes. Epistasis studies with ppGpp0 mutants deleted also for either mfd or uvrD revealed additive effects of deleting mfd but not uvrD, which would suggest that ppGpp and UvrD affect the same mechanism, as if the backtracking function known for UvrD is likely to be the ppGpp contribution as well.
The transcription elongation factors GreA and GreB are even better known for their backtracking functions. When greA and greB are deleted, the otherwise sensitive ppGpp0 cells become resistant to DNA damage. DksA is another transcription factor often required for (p)ppGpp regulation. We showed previously that deletion of both greAB and dksA are (synthetic) lethals. It is consistent that deleting dksA sensitizes wild-type cells to damage, while overexpressing DksA makes them more resistant. These many inferences could be verified at the level of in vitro transcription with purified components. Adding UvrD potentiated backtracking, visualized as enhanced pausing in single-round runoff transcripts. The effect was further enhanced by adding ppGpp with or without DksA but not by adding DksA alone. Others have reported a modest cleft opening of the DNA clamp of RNA polymerase (sometimes called a crab claw) when ppGpp is added. It is plausible that this effect of ppGpp might weaken the grip of polymerase on the DNA template and favor backtracking. Such a mechanism could explain how ppGpp might couple transcription to DNA repair. Again in vitro studies provide supportive evidence. RNAP can be assembled in elongation complexes at a fixed position then probed with exonuclease III digestion to observe that the presence of ppGpp shifts the position backward. Consistent changes could also be found in vivo footprints with or without ppGpp as well as by deleting or overexpressing uvrD. Finally, effects of ppGpp could be shown to be mimicked by an RNA polymerase mutant (RpoB*35, H1244Q), previously found to phenocopy several regulatory effects of ppGpp. The proposed model for ppGpp facilitation of transcription-coupled repair involves transient ppGpp accumulation, which may be of advantage to avoid possible complications of more permanent effects, such as excessive slippage or collisions with replication forks. Exactly how DNA damage provokes ppGpp accumulation and why it is transient remains a puzzle. Future approaches might be to measure the effect of relA or spoT mutants on ppGpp accumulation during exposures to DNA–damaging agents. These genes are known to mediate specific sensing systems that provoke ppGpp.
Publications
- Gaca AO, Kudrin P, Colomer-Winter C, Beljantseva J, Liu K, Anderson B, Wang JD, Rejman D, Potrykus K, Cashel M, Hauryliuk V, Lemos JA. From (p)ppGpp to (pp)pGpp: characterization of regulatory effects of pGpp synthesized by the small alarmone synthetase of Enterococcus faecalis. J Bacteriol 2015; 197(18):2908-2919.
- Kamarthapu V, Epshtein V, Benjamin B, Proshkin S, Mironov A, Cashel M, Nudler E. ppGpp couples transcription to DNA repair in E. coli. Science 2016;352:993-936.
- Potrykus K, Cashel M. Preferential cellular accumulation of ppGpp or pppGpp in Escherichia col. In: de Brujin FJ, ed. Stress and Environmental Regulation of Gene Expression and Adaptation in Bacteria. John Wiley and Sons, Inc. 2016;7:1-8.
Collaborators
- Katarzyna Potrykus, PhD, University of Gdansk, Gdansk, Poland
- Evgeny Nudler, PhD, New York University School of Medicine, New York, NY
- Agnieszka Szalewska-Palasz, PhD, University of Gdansk, Gdansk, Poland
Contact
For more information, email cashel@mail.nih.gov or visit http://smr.nichd.nih.gov.