Childhood Neurodegenerative Lysosomal Storage Disorders
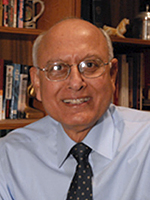
- Anil B. Mukherjee, MD, PhD, Head, Section on Developmental Genetics
- Zhongjian (Gary) Zhang, MD, PhD, Staff Scientist
- Maria B. Bagh, PhD, Research Fellow
- Arnab Datta, PhD, Visiting Fellow
- Tamal Sadhukhan, PhD, Visiting Fellow
- Ashleigh Bouchelion, PhD, MD Student
- Sondra W. Levin, MD, Adjunct Scientist
The Section on Developmental Genetics conducts both laboratory and clinical investigations into the neurodegenerative lysosomal storage disorders (LSDs) primarily affecting children. Our current research focuses on understanding the molecular mechanism(s) of pathogenesis of a group of hereditary childhood neurodegenerative LSDs called neuronal ceroid lipofuscinoses (NCLs), the most common of which is known as Batten disease. Mutations in at least 13 different genes underlie various types of NCLs, and the list continues to grow. Currently, there is no effective treatment for any of the NCL types. Among all the NCLs, infantile NCL (INCL) and congenital NCL (CNCL) are the most devastating diseases. INCL is a neurodegenerative, autosomal recessive LSD caused by mutations in the CLN1 gene, which encodes palmitoyl-protein thioesterase-1 (PPT1), a lysosomal depalmitoylating enzyme. Numerous proteins, especially in the brain, undergo palmitoylation, which is a post-translational modification of polypeptides in which a long-chain saturated fatty acid (predominantly palmitate) is attached to specific cysteine residues. Palmitoylation (also called S-acylation) of proteins plays important roles in signal transduction pathways, and constitutive and controlled turnover of palmitate regulates membrane association and intracellular trafficking of signaling GTPases. Moreover, numerous receptors, transporters, and ion channels are S-acylated with diverse functional consequences, including protein-protein interactions, stability, and the regulation of assembly and trafficking. Palmitoylation is catalyzed by DHHC (Asp-His-His-Cys)-palmitoyl-acyltransferases (PATs), and the removal of palmitate (depalmitoylation) is catalyzed by palmitoyl-protein thioesterases (PPTs).
While S-acylation regulates the function of many important proteins requiring membrane anchorage, the proteins must also be depalmitoylated for recycling or for degradation by lysosomal hydrolases. Therefore, dynamic palmitoylation (palmitoylation-depalmitoylation), essential for steady-state membrane localization and signaling by many proteins, requires the interplay of both the DHHC-PATs and the PPTs. Several years ago, it was discovered that mutations in a lysosomal PPT1 cause accumulation of palmitoylated proteins (constituents of ceroid) in lysosomes, leading to INCL. However, the precise molecular mechanism of INCL pathogenesis remains largely unclear. Children afflicted with INCL are normal at birth, but exhibit signs of myoclonus, psychomotor retardation, and seizures followed by progressive loss of developmental milestones by 11 to 18 months of age. By two years of age, these children are completely blind as a result of retinal degeneration. At around four years of age, they fail to show any electrical activity manifested by an isoelectric electroencephalogram (EEG), including visual evoked potentials, and remain in a persistent vegetative state for several more years until death. These grim facts underscore the desirability of developing an effective therapy for this disease. Our efforts are thus directed to first understand the molecular mechanism(s) of pathogenesis through innovative laboratory investigations and, second, to apply the knowledge gained from our laboratory investigations to develop novel therapeutic strategies for INCL (CLN1 disease), juvenile NCL (JNCL or CLN3 disease), and possibly other types of Batten disease.
In our laboratory studies on INCL and JNCL, we use cultured cells from patients with these diseases as well as the mouse models (Cln1–/– and Cln3–/– mice), which recapitulate virtually all clinical and pathological features of INCL and JNCL, respectively. During the past several years, we discovered that PPT1 deficiency causes endoplasmic-reticulum (ER) and oxidative stress, which at least in part contribute to the neuropathology in INCL. Moreover, we delineated a mechanism by which PPT1 deficiency may disrupt the recycling of the synaptic vesicles (SVs), causing progressive loss of the SV pool size that is required for maintaining uninterrupted neurotransmission at nerve terminals. We also developed a noninvasive method, using MRI and MRS (magnetic resonance spectroscopy), to evaluate the progression of neurodegeneration in Ppt1–/– mice. The method permits repeated evaluations of potential therapeutic agents in treated animals. In addition, in collaboration with the NEI, we are conducting studies to determine whether electro-retinography can be used to assess the progressive retinal deterioration in Cln1–/– as well as in Cln1–knock-in (KI) mice, which carry the most common nonsense mutation found in the INCL patient population in the US. We also discovered that the blood-brain barrier is disrupted in Ppt1–/– mice and that this pathology is ameliorated by treatment with resveratrol, which has anti-oxidant properties. More recently, we identified and characterized a non-toxic, thioesterase-mimetic, antioxidant small molecule, N-(tert-Butyl) hydroxylamine (NtBuHA), which mediates ceroid depletion, preserves motor function, and modestly extends lifespan of Cln1–/– mice. Our results suggest that thioesterase-mimetic small molecules such as NtBuHA are potential therapeutic targets for INCL. More recently, we discovered that cathepsin D (CD) deficiency in lysosomes is a common pathogenic link between INCL (CLN1 disease) and congenital NCL (CNCL) or CLN10 disease. Our current efforts are aimed at determining the common pathogenic link(s) among all NCLs.
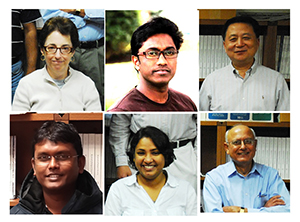
Click image to enlarge.
Mukherjee Laboratory Staff
Upper Left to Right: Sondra W. Levin, MD; Abhilash P. Appu, PhD; Gary Zhang, MD, PhD
Lower Left to Right: Tamal Sadhukhan, PhD; Maria B. Bagh, PhD; Anil B. Mukherjee, MD, PhD
Discovery of a common pathogenic link between INCL and CNCL
In multicellular organisms, the lysosome is the major degradative organelle responsible for disposing of the damaged macromolecules and organelles in the cell. It has been reported that impaired lysosomal degradative capability leads to pathogenesis of many neurodegenerative disorders, including LSDs. As noted above, neurodegeneration is a devastating manifestation in the majority of the more than 50 LSDs. Moreover, lysosomal degradative capability has been reported to be impaired in several late-onset neurodegenerative diseases such as Alzheimer’s, Huntington’s, and Parkinson’s. Cathepsin D (CD) is a major lysosomal aspartic protease in lysosomes. Lysosomal CD activity catalyzes degradation and clearance of exogenous as well as endogenous macromolecules and damaged organelles delivered to the lysosome. Intracellular accumulation of undegraded long-lived proteins and other macromolecules leads to the pathogenesis of many neurodegenerative disorders. Paradoxically, both CD overexpression and CD deficiency have been reported to underlie neurodegenerative diseases. However, despite intense studies, this paradox has, until now, remained poorly understood.
While inactivating mutations in the CLN1 gene encoding palmitoyl-protein thioesterase-1 (PPT1) cause INCL, mutations in the CLN10/CTSD gene encoding CD underlie CNCL. We sought to determine whether there is a pathogenic link between INCL and CNCL. The synthesis of CD occurs in the ER as a pre-propeptide with a molecular mass of about 50 kDa. The cleavage of the leader peptide in the ER generates the 48 kDa precursor of mature-CD (pro-CD). In the Golgi complex, attachment of mannose 6-phosphate to pro-CD facilitates the protein's binding to endosomal/lysosomal sorting receptors. The receptor-ligand complexes then exit the trans-Golgi network in clathrin-coated intermediates and fuse with the endosomal system. The low pH of the late endosomal lumen facilitates dissociation of the receptor-ligand complexes and allows the ligand (i.e., pro-CD) to be delivered to lysosome. The pro-CD then undergoes further proteolytic cleavage by cathepsin B (CB) and cathepsin L (CL), which generate, respectively, the 31 and 14 kDa fragments, non-covalent dimerization of which constitutes the mature, catalytically active CD. We used Cln1–/–/Ppt1–/– mice, which recapitulate virtually all clinical and pathological features of INCL, to test for a pathogenic link between INCL and CNCL. Our results show that despite Cln10/Ctsd overexpression, defective processing of pro-CD to mature CD in lysosome leads to lysosomal CD deficiency causing neuropathology in INCL. Given that CD deficiency underlies CNCL, we propose that CD deficiency in the lysosome is the link between INCL and CNCL.
MR spectroscopy to evaluate disease progression in INCL
The pathological findings for INCL include accumulation of intracellular autofluorescent material (ceroid), rapidly progressive brain atrophy resulting predominantly from loss of cortical neurons in the cerebrum, and neuro-inflammatory findings. The accumulation of ceroid is a characteristic pathological finding in all NCLs. Although the components and ultrastructure of the storage material vary across the different types of NCLs, electron-microscopic analyses of the brain and other tissues from INCL patients show characteristic granular osmiophilic deposits (GRODs).
Previous studies in cell cultures derived from INCL patients demonstrated that phosphocysteamine had a beneficial effect on the cell culture, resulting in depletion of intra-lysosomal ceroid deposits and suppression of apoptosis. N-acetylcysteine, a potent antioxidant, has been reported to have beneficial effects on other neurodegenerative diseases. Because INCL is a rare disease (1 in over 100,000 births) with a short life expectancy, recruitment of a large study group is impractical; this consideration, together with promising results from cell-culture experiments, led to a “compassionate use” type of experimental design in which all patients received treatment with cysteamine bitartrate (Cystagon) and N-acetylcysteine (Mucomyst), without a control group, with the intention of comparing to the natural history and to future treatment interventions using quantifiable measures. These included behavioral and developmental assessments, EEG, ERG, MRI–derived brain volume measurements, and quantification of intra-lysosomal ceroid deposits. Towards the end of the study's recruitment period, quantitative MRS was added to the quantifiable measures. Recently, an overview of the study results was published (Reference 4), and details the quantitative MRS findings are listed below.
As part of the pilot study to evaluate treatment benefits of cysteamine bitartrate and N-acetylcysteine, we quantitatively measured brain metabolite levels using MRS. A subset of two patients from a larger treatment and follow-up study underwent serial quantitative single-voxel MRS examinations of five anatomical sites. Three echo times were acquired in order to estimate metabolite T2 [quantification of the absolute concentration of metabolites using long-echo-time (TE) acquisition schemes]. Measured metabolite levels included a correction for partial volume of cerebrospinal fluid. We compared INCL patients with a reference group composed of asymptomatic and minimally symptomatic Niemann-Pick disease type C patients. In INCL patients, N-acetylaspartate (NAA) was abnormally low at all locations upon initial measurement and further declined throughout the follow-up period. In the cerebrum (affected early in the disease course) choline and myo-inositol levels were initially elevated and fell during the follow-up period, whereas in the cerebellum and brainstem (affected later) choline and myo-inositol levels were initially normal and rose subsequently. Choline and myo-inositol levels in our patients are consistent with patterns of neuro-inflammation observed in two INCL mouse models. Low, persistently declining NAA was expected based on the progressive, irreversible nature of the disease. Progression of metabolite levels in INCL has not been previously quantified; therefore the results of this study serve as a reference for quantitative evaluation of future therapeutic interventions.
Brain volume measurements in INCL by MRI
Neurodegeneration is the devastating manifestation in most of the more than 50 known lysosomal storage disorders. Mutations in at least 13 different genes cause various types of NCLs. Among these genes, CLN1, CLN2, CLN10, and CLN13 encode lysosomal enzymes; CLN4 and CLN14 encode peripherally associated cytoplasmic proteins; CLN5 encodes a soluble lysosomal protein; CLN11 encodes a protein in the secretory pathway; and several transmembrane proteins with varying subcellular localizations are encoded by CLN3, CLN6, CLN7, CLN8, and CLN12.
To evaluate the treatment benefits of the combination of cysteamine bitartrate and N-acetylcysteine, we made serial measurements of patients’ brain volumes with MR imaging. Ten patients with infantile neuronal ceroid lipofuscinosis participating in a treatment/follow-up study underwent brain MR imaging that included high-resolution T1-weighted images. After manual placement of a mask delineating the surface of the brain, a maximum-likelihood classifier was applied to determine total brain volume, further subdivided as cerebrum, cerebellum, brain stem, and thalamus. Patients’ brain volumes were compared with those of a healthy population. Major subdivisions of the brain followed similar trajectories with different timing. The cerebrum demonstrated early, rapid volume loss and may never have been normal postnatally. The thalamus dropped out of the normal range around six months of age, the cerebellum around two years of age, and the brain stem around three years of age. Rapid cerebral volume loss was expected on the basis of previous qualitative reports. Because our study did not include a non-treatment arm and because progression of brain volumes in infantile neuronal ceroid lipofuscinosis has not been previously quantified, we could not determine whether our intervention had a beneficial effect on brain volumes. However, the level of quantitative detail in this study allows it to serve as a reference for evaluation of future therapeutic interventions.
Mechanism underlying the lysosomal acidification defect in the INCL mouse model
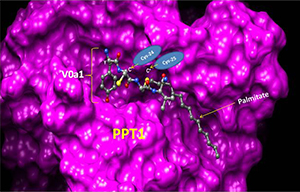
Click image to enlarge.
Proposed molecular model explaining how Ppt1 may catalyze V0a1 depalmitoylation on the lysosomal membrane
The model is generated by using the YCCV (tyrosine/cysteine/cysteine/valine) peptide in palmitoylated V0a1 docked into the Ppt1 active site located in its hydrophobic groove. It suggests that S-palmitoylated Cys-25, but not Cys-24, may interact with the catalytic site of Ppt1 to facilitate V0a1's depalmitoylation. The crystal structure of Ppt1 was resolved, which demonstrated the presence of the catalytic site in the hydrophobic core, through which substrates are depalmitoylated. Taken from Bellizzi JJ et al. Proc Natl Acad Sci USA 2000;97:4573.
In eukaryotic organisms, the lysosome is the primary organelle for intracellular digestion. It contains more than 50 hydrolases, which require an acidic pH for optimal degradative function. Thus, lysosomal acidification is of fundamental importance in the degradation of macromolecules of intra- and extra-cellular origin that are delivered to the lysosome. Moreover, it has been reported that dysregulation of lysosomal acidification contributes to pathogenesis in virtually all lysosomal storage disorders (LSDs), including several NCLs. Furthermore, defective regulation of lysosomal pH has also been reported in common neurodegenerative diseases such as Alzheimer’s and Parkinson’s. However, despite intense studies, the mechanism(s) underlying the lysosomal acidification defect remains largely unclear.
Lysosomal acidification is regulated by vacuolar ATPase (v-ATPase), a multi-subunit protein complex composed of the cytosolic V1-sector and the lysosomal membrane-anchored V0-sector. Reversible assembly of V1/V0 sectors on the lysosomal membrane maintains functionally active v-ATPase, the proton pump of the cell, which regulates lysosomal acidification.
Palmitoylation (also called S-acylation) is a reversible post-translational modification in which a long-chain fatty acid (predominantly palmitate) is attached to specific cysteine residues in polypeptides via thioester linkage. In the mammalian genome, 23 genes encode palmitoyl-acyl-transferases (PATs), which are evolutionarily conserved, cysteine-rich proteins containing Asp-His-His-Cys (DHHC) domains that catalyze palmitoylation. In contrast, there are four thioesterases that have been characterized, two of which are cytosolic [e.g., acyl-protein thioesterase-1 (Apt1) and Apt2] and two [e.g., palmitoyl-protein thioesterase-1 (PPT1) and PPT2] are localized to the lysosome. Dynamic palmitoylation (palmitoylation-depalmitoylation), requiring coordinated action of both the DHHC-PATs and PPTs, maintain steady-state membrane localization and function of numerous important proteins, especially in the brain. By catalyzing depalmitoylation, thioesterases also facilitate recycling or degradation of proteins that undergo palmitoylation.
We tested the hypothesis that one or more subunits of v-ATPase requires S-palmitoylation for endosomal sorting, trafficking, and reversible assembly of V1/V0 on the lysosomal membrane, which is essential for regulating lysosomal pH, and that Ppt1 deficiency disrupts v-ATPase activity, impairing its proton transport function, thereby dysregulating acidification of lysosomal lumen. Our results show that the lysosomal membrane–anchored V0a1 subunit of v-ATPase undergoes S-palmitoylation, which is required for its sorting and trafficking to the lysosomal membrane. The process appears to be defective in Ppt1–deficient Cln1–/– mice. Notably, we demonstrated that treatment of these mice with the thioesterase (Ppt1)-mimetic small molecule NtBuHA restores v-ATPase activity and rescues defective the lysosomal acidification phenotype.
Publications
- Bagh MB, Peng S, Chandra G, Zhang Z, Singh SP, Pattabiraman N, Liu A, Mukherjee AB. Misrouting of v-ATPase subunit V0a1 dysregulates lysosomal acidification in a neurodegenerative lysosomal storage disease model. Nat Commun 2016;in press.
- Baker EH, Levin SW, Zhang Z, Mukherjee AB. MRI brain volume measurements in infantile neuronal ceroid lipofuscinosis. Am J Neuroradiol 2017;38(2):376-382.
- Chandra G, Bagh MB, Peng S, Saha A, Sarkar C, Moralle M, Zhang Z, Mukherjee AB. Cln1 gene disruption in mice reveals a common pathogenic link between two of the most lethal childhood neurodegenerative lysosomal storage disorders. Hum Mol Genet 2015;14:5416-5432.
- Baker EH, Levin SW, Zhang Z, Mukherjee AB. Evaluation of disease progression in INCL by MR spectroscopy. Ann Clin Transl Neurol 2015;2:797-809.
Collaborators
- Eva Baker, MD PhD, Radiology and Imaging Sciences, Clinical Center, NIH, Bethesda, MD
- Chris J. McBain, PhD, Section on Cellular and Synaptic Physiology, NICHD, Bethesda, MD
- Kenneth Pelky, PhD, Section on Cellular and Synaptic Physiology, NICHD, Bethesda, MD
- Ling-Gang Wu, PhD, Synaptic Transmission Section, NINDS, Bethesda, MD
Contact
For more information, email mukherja@exchange.nih.gov or visit irp.nih.gov/pi/anil-mukherjee.