Transcriptional and Translational Regulatory Mechanisms in Nutrient Control of Gene Expression
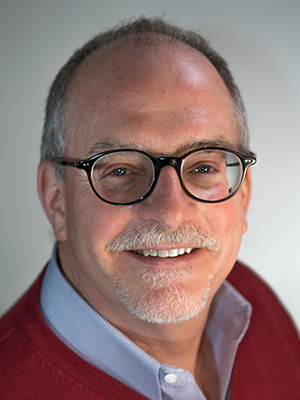
- Alan G. Hinnebusch, PhD, Head, Section on Nutrient Control of Gene Expression
- Hongfang Qiu, PhD, Staff Scientist
- Neelam Sen, PhD, Research Fellow
- Suna Gulay, PhD, Postdoctoral Fellow
- Neha Gupta, PhD, Postdoctoral Fellow
- Anil Thakur, PhD, Postdoctoral Fellow
- Jyothsna Visweswaraiah, PhD, Postdoctoral Fellow
- Fnu Yashpal, PhD, Postdoctoral Fellow
- David Young, PhD, Postdoctoral Fellow
- Quira Zeiden, PhD, Postdoctoral Fellow
- Jinsheng Dong, PhD, Senior Research Assistant
- Fan Zhang, MS, Senior Research Assistant
- Laura Marler, BS, Graduate Student
We study the fundamental mechanisms involved in the assembly and function of translation initiation complexes for protein synthesis, using yeast as a model system to exploit its powerful combination of genetics and biochemistry. The translation initiation pathway produces an 80S ribosome bound to mRNA with methionyl initiator tRNA (tRNAi) base-paired to the AUG start codon. The tRNAi is recruited to the small (40S) subunit in a ternary complex (TC) with GTP–bound eIF2 to produce the 43S preinitiation complex (PIC) in a reaction stimulated by eIFs 1, 1A, 3, and 5. The 43S PIC attaches to the 5′ end of mRNA, facilitated by cap-binding complex eIF4F (comprising eIF4E, eIF4G, and the RNA helicase eIF4A) and poly(A)-binding protein (PABP) bound to the poly(A) tail, and scans the 5′ untranslated region (UTR) for the AUG start codon. Scanning is promoted by eIFs 1 and 1A, which induce an open conformation of the 40S and rapid TC binding in a conformation suitable for scanning successive triplets entering the ribosomal P site (P-out), and by eIF4F and other RNA helicases, such as Ded1, that remove secondary structure in the 5′ UTR. AUG recognition evokes tighter binding of TC in the P-in state and irreversible GTP hydrolysis by eIF2, dependent on the GTPase–activating protein (GAP) eIF5, releasing eIF2-GDP from the PIC with tRNAi remaining in the P site. Joining of the 60S subunit produces the 80S initiation complex ready for protein synthesis. Our current aims in this research area are to: 1) elucidate functions of eIF1, eIF1A, eIF2, and 40S proteins in TC recruitment and start codon recognition; 2) identify functions of eIF4G, eIF4B/Tif3, and Ded1 in mRNA activation, 48S PIC assembly, and scanning in vivo; 3) uncover the mechanisms of translational repression by Scd6, Pat1, Dhh1 and Khd1; and 4) elucidate the mechanism of ribosome recycling at stop codons in vivo.
We also analyze the regulation of amino acid–biosynthetic genes in budding yeast as a means of dissecting fundamental mechanisms of transcriptional control of gene expression. Transcription of these genes is coordinately induced by the activator Gcn4 during amino acid limitation owing to induction of Gcn4 at the translational level. The eviction of nucleosomes that occlude promoter DNA sequences and block access by RNA polymerase is thought to be a rate-limiting step for transcriptional activation. Previous studies implicated certain histone chaperones, ATP–dependent chromatin remodeling complexes, or histone acetyltransferase complexes in eviction of promoter nucleosomes at certain yeast genes, but it was unclear whether these co-factors function at Gcn4 target genes. Our current aim is to identify the full set of co-factors that participate in promoter nucleosome eviction at Gcn4 target genes, their involvement in this process genome-wide, and the transcriptional consequences of defective nucleosome eviction.
Structures of yeast preinitiation complexes reveal conformational changes from mRNA scanning to start-codon recognition.

Click image to enlarge.
Model of structural rearrangements in the eukaryotic translation preinitiation complex (PIC) involved in start codon recognition
(i) Binding of eIF1 and eIF1A to the 40S evokes an open conformation conducive to rapid TC binding, forming the 43S PIC. (ii) The 43S scans the mRNA 5′ UTR, with the anticodon stem loop (ASL) of Met-tRNAi not fully engaged with the P site (POUT) but capable of sampling triplets for complementarity to the anticodon as they enter the P site. The GAP domain in the eIF5-NTT (5N) stimulates GTP hydrolysis to produce GDP·Pi, but release of Pi is impeded. The unstructured NTT of eIF2b interacts with eIF1 to stabilize this open conformation of the PIC. (iii) Base-pairing between the ASL and AUG codon promotes movement of the tRNA from the POUT to PIN state, displacing eIF1 from its location near the P site to a new 40S binding site that overlaps with the eIF5-CTD binding site. This movement of eIF1 eliminates its interaction with the eIF2b NTT, and the latter interacts tightly with the eIF5-CTD instead. (iv) eIF1 dissociates from the 40S subunit to stabilize the closed, scanning-incompatible conformation of the 40S subunit. Ejection of eIF1 allows the eIF5-NTT to dissociate from the G domain of eIF2g and bind to the 40S subunit at a location overlapping the eIF1 binding site, facilitating a functional interaction with the eIF1A-CTT that triggers release of Pi from eIF2-GDP, and blocking reassociation of eIF1 with the 40S subunit. (Published in Hinnebusch AG. The scanning mechanism of eukaryotic translation initiation. Annu Rev Biochem 2014;83:779.)
It is thought that the scanning PIC assumes an open conformation and that AUG recognition evokes a closed state that arrests scanning with more stable tRNAi binding (P-in state), with attendant displacement of the eIF1A-CTT (C-terminal tail) from the P site and dissociation of eIF1 from the 40S subunit. In collaboration with Venki Ramakrishnan's and Jon Lorsch's groups, we obtained cryo-EM reconstructions of yeast PICs that represent different stages of the initiation pathway, at 3.5–6.1 Å resolution. These include 40S-eIF1-eIF1A complexes and partial yeast 48S PICs in open and closed conformations (py48S-open and py48-closed). The structures yield considerable new information about conformational changes in the transition from scanning to AUG selection. The py48S-open complex, formed using mRNA with AUC start codon, reveals an upward shift of the 40S head that widens the mRNA entry channel and opens its latch—consistent with our eIF1A/Fe(II)–BABE cleavage data—which should facilitate mRNA insertion into the binding cleft to form the scanning PIC. Moreover, the P site is widened and lacks tRNAi contacts with the 40S body present in canonical 80S-tRNAi complexes. Formed with mRNA(AUG), py48S-closed reveals downward head movement that closes the latch, clamps the mRNA into the binding cleft, and fully encloses tRNAi in the P site. The eIF1A N-terminal tail (NTT) interacts with the AUG:anticodon duplex, consistent with its role in stabilizing P-in. As a prelude to eIF1 dissociation from the 40S subunit, eIF1 is repositioned on the 40S and deformed to prevent a clash with tRNAi. Both py48S-open and -closed complexes reveal eIF2beta and portions of the eIF3 complex. The eIF3 trimeric subcomplex eIF3b-CTD/eIF3i/eIF3g-NTT resides on the subunit-interface surface of the 40S and appears to lock mRNA into the 40S binding cleft. Exclusively in py48S-open, eIF2beta interacts with tRNAi and segments of eIF1 and eIF1A, which should stabilize binding of TC and eIF1 to the scanning PIC prior to AUG recognition. Indeed, we showed that mutations at the eIF2beta:eIF1 interface increase aberrant recognition of UUG codons in vivo—the expected consequence of shifting the system from the open to closed conformations—thus establishing the physiological relevance of the py48S-open and py48S-closed structures.
eIF4B and DEAD-box RNA helicases Ded1 and eIF4A preferentially stimulate translation of long mRNAs with structured 5′ UTRs and low closed-loop potential but with weak dependence on eIF4G.
The RNA helicases eIF4A and Ded1 are believed to resolve mRNA secondary structures that impede ribosome attachment to the mRNA and scanning to the start codon, but whether they perform distinct functions in vivo was poorly understood. We compared the effects of mutations in Ded1 or eIF4A on translational efficiencies (TEs) by ribosome profiling. Despite similar reductions in bulk translation, inactivation of Ded1 substantially reduced the relative TEs of over 600 mRNAs, whereas inactivation of eIF4A similarly affected fewer than 40 mRNAs. Ded1–dependent mRNAs show greater than average 5′ UTR length and propensity for secondary structure, implicating Ded1 in scanning though structured 5′ UTRs. Thus, it appears that Ded1 is critically required for scanning through secondary structures in 5′ UTRs, while eIF4A promotes a step of initiation common to nearly all mRNAs, such as ribosome attachment. Ribosome profiling of a mutant lacking eIF4B showed that eliminating eIF4B preferentially impacts mRNAs with long structured 5′ UTRs and reduces the relative TEs of many more genes than does inactivation of eIF4A. The findings support an eIF4A–independent role for eIF4B in addition to its known function as eIF4A cofactor. Mutations in eIF4B, eIF4A, and Ded1 also preferentially impair translation of longer mRNAs in a manner mitigated by the ability to form closed-loop mRNPs via eIF4F-Pab1 association, suggesting cooperation between closed-loop assembly and eIF4B/helicase functions in stimulating initiation.
Rli1/ABCE1 recycles terminating ribosomes and controls translation reinitiation in 3′ UTRs in vivo.
Ribosome recycling is the final phase of protein synthesis, following release of the completed polypeptide chain from the ribosome at the stop codon. ABCE1 (ATP binding cassette subfamily E member 1; yeast Rli1) has been shown to catalyze removal of the 60S subunit from post-termination complexes in vitro but not to be crucial for recycling in vivo. In collaboration with Rachel Green’s lab, we used ribosome profiling to show that depletion of Rli1 in yeast evokes accumulation of 80S ribosomes at stop codons and in the adjoining 3′ UTRs of most genes. Predicted 3′ UTR translation was detected by Western analysis and mass spectrometry of epitope-tagged strains, and their small sizes indicate a reinitiation mechanism whereby, following polypeptide release at the stop codon, unrecyled 80S ribosomes resume translation at a nearby sense codon. Thus, Rli1 is crucial for ribosome recycling in vivo and to prevent inappropriate 3′ UTR translation.
Genome-wide cooperation by histone acetyl transferase Gcn5, remodeler SWI/SNF, and chaperone Ydj1 in promoter nucleosome eviction and transcriptional activation
We investigated the mechanism of promoter nucleosome eviction by analyzing the effects of mutations in one or more chromatin remodeling complexes, histone acetyltransferase (HAT) complexes, or histone chaperone on eviction of histone H3 at the hundreds of genes in the Gcn4 transcriptome during their induction by Gcn4. By conventional chromatin immunoprecipitation analysis (ChIP) of four canonical Gcn4 target genes, ARG1, HIS4, ARG4, and CPA2, we excluded a requirement for several co-factors implicated previously at other genes (e.g., Asf1, Nap1, RSC) and implicated the remodeler SWI/SNF (Snf2), HAT Gcn5, and Hsp70 co-chaperone Ydj1 in nucleosome eviction at these Gcn4 target genes. Expanding our analysis genome-wide by H3 ChIP-Seq, we found that Snf2, Gcn5, and Ydj1 collaborate in evicting H3 from the –1 and +1 promoter nucleosomes and intervening nucleosome-depleted region (NDR) at a large fraction of the Gcn4 transcriptome. We found that the three cofactors function similarly at virtually all yeast promoters. Surprisingly, however, defective H3 eviction in co-factor mutants was coupled with reduced transcription (Pol II densities measured by Rpb3 ChIP-Seq) for only a subset of genes, which included the induced Gcn4 transcriptome and the most highly expressed subset of constitutively expressed yeast genes. In fact, the most weakly expressed genes displayed higher transcription than other genes in response to global attenuation of nucleosome eviction. We thus established that steady-state eviction of promoter nucleosomes is required for maximal transcription of highly expressed genes and that Gcn5, Snf2, and Ydj1 function broadly in this step of gene activation, but we discovered unexpectedly that some other aspect of transcriptional activation is more generally rate-limiting for transcription of most genes in amino acid–deprived yeast.
Publications
- Zhang F, Saini AK, Shin BS, Nanda J, Hinnebusch AG. Conformational changes in the P site and mRNA entry channel evoked by AUG recognition in yeast translation preinitiation complexes. Nucleic Acids Res 2015;43:2293-2312.
- Sen ND, Zhou F, Ingolia NT, Hinnebusch AG. Genome-wide analysis of translational efficiency reveals distinct but overlapping functions of yeast DEAD-box RNA helicases Ded1 and eIF4A. Genome Res 2015;25:1196-1220.
- Young DJ, Guydosh NR, Zhang F, Hinnebusch AG, Green R. Rli1/ABCE1 recycles terminating ribosomes and controls reinitiation in 3’UTRs in vivo. Cell 2015;162:872-884.
- Llacer JL, Hussain T, Marler L, Aitken CE, Thakur A, Lorsch JR, Hinnebusch AG. Conformational differences between open and closed states of the eukaryotic translation initiation complex. Mol Cell 2015;59:399-412.
- Qiu H, Chereji RV, Hu C, Cole HA, Rawal Y, Clark DJ, Hinnebusch AG. Genome-wide cooperation by HAT Gcn5, remodeler SWI/SNF, and chaperone Ydj1 in promoter nucleosome eviction and transcriptional activation. Genome Res 2016;26:211-225.
- Sen ND, Zhou F, Harris MS, Ingolia NT, Hinnebusch AG. eIF4B stimulates translation of long mRNAs with structured 5' UTRs and low closed-loop potential but weak dependence on eIF4G. Proc Natl Acad Sci USA 2016;113:10464-10472.
Collaborators
- Katsura Asano, PhD, Kansas State University, Manhattan, KS
- David Clark, PhD, Section on Chromatin and Gene Expression, NICHD, Bethesda, MD
- Rachel Green, PhD, The Johns Hopkins University School of Medicine, Baltimore, MD
- Nicholas Ingolia, PhD, University of California Berkeley, Berkeley, CA
- Jon Lorsch, PhD, Laboratory on the Mechanism and Regulation of Protein Synthesis, NICHD, Bethesda, MD
- Venkatraman Ramakrishnan, PhD, MRC Laboratory of Molecular Biology, Cambridge, UK
- Gerhard Wagner, PhD, Harvard Medical School, Boston, MA
Contact
For more information, email hinnebua@mail.nih.gov or visit http://sncge.nichd.nih.gov.