Cell Cycle Regulation in Oogenesis
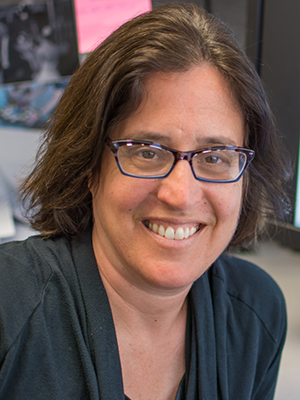
- Mary Lilly, PhD, Head, Section on Gamete Development
- Lucia Bettedi, PhD, Visiting Fellow
- Weili Cai, PhD, Visiting Fellow
- Youheng Wei, PhD, Visiting Fellow
- Kuikwon Kim, MS, Technician
- Megan Bannon, BS, Postbaccalaureate Fellow
- Elena Ghanaim, BS, Postbaccalaureate Fellow
- Nicholas Johnson, BS, Postbaccalaureate Fellow
The long-term goal of our laboratory is to understand how the cell-cycle events of meiosis are coordinated with the developmental events of gametogenesis. Chromosome mis-segregation during female meiosis is the leading cause of miscarriages and birth defects in humans. Recent evidence suggests that many meiotic errors occur downstream of defects in oocyte growth and/or the hormonal signaling pathways that drive differentiation of the oocyte. Thus, an understanding of how meiotic progression and gamete differentiation are coordinated during oogenesis is essential to studies in both reproductive biology and medicine. We use the genetically tractable model organism Drosophila melanogaster to examine how meiotic progression is instructed by the developmental and metabolic program of the egg.
In mammals, studies on the early stages of oogenesis face serious technical challenges in that entry into the meiotic cycle, meiotic recombination, and the initiation of the highly conserved prophase I arrest all occur during embryogenesis. By contrast, in Drosophila these critical events of early oogenesis all take place continuously within the adult female. Easy access to the early stages of oogenesis, coupled with available genetic and molecular genetic tools, makes Drosophila an excellent model for studies on meiotic progression and oocyte development.
To understand the regulatory inputs that control early meiotic progression, we are working to determine how the oocyte initiates and then maintains the meiotic cycle within the challenging environment of the ovarian cyst. Our studies focus on the following questions, which are relevant to the development of all animal oocytes. What strategies does the oocyte use to protect itself against inappropriate DNA replication? How does the oocyte inhibit mitotic activity before meiotic maturation and the full growth and development of the egg? How does cell-cycle and metabolic status within the ovarian cyst influence the differentiation of the oocyte? To answer these questions, we have undertaken studies to determine the basic cell-cycle program of the developing ovarian cyst.
The GATOR complex: integrating developmental and metabolic signals in oogenesis
We are interested in how metabolism influences oocyte growth, development, and quality. Target of Rapamycin Complex 1 (TORC1) is a primary regulator of cell growth and metabolism, which responds to several upstream signals, including nutrient availability, energy status, and growth factors. In the past year, we defined the role of the GATOR complex in the regulation of metabolic homeostasis, autophagic flux, endomembrane dynamics, and oocyte development. Our findings define Drosophila as an excellent model for the genetic dissection of the GATOR complex and its role in preventing the developmental defects and pathologies associated with deregulated TORC1 activity. Additionally, they clearly establish a conserved role for the GATOR complex in the regulation of meiotic progression and gametogenesis.
The GATOR complex comprises two sub-complexes. The GATOR1 complex inhibits TORC1 activity in response to amino acid starvation by preventing the targeting of TORC1 to lysosomes, where it is activated by the GTP–binding protein Rheb. GATOR1 is a trimeric protein complex, consisting of the proteins Nprl2, Nprl3, and Iml1. Recent evidence, from yeast and mammals, indicates that the components of the GATOR1 complex function as GTPase–activating proteins (GAP) that inhibit TORC1 activity by inactivating the Rag GTPases, which are required for TORC1 lysosomal recruitment. Notably, Nprl2 and Iml1 are tumor suppressor genes while mutations in Iml1, known as DEPDC5 in mammals, are the leading cause of hereditary epilepsy.
The GATOR2 complex comprises five proteins: Seh1, Sec13, Mio, Wdr24, and Wdr59. Our work, as well as work from others, found that GATOR2 complex components activate TORC1 by opposing the TORC1–inhibitory activity of GATOR1. Intriguingly, computational analysis indicates that Mio and Seh1, as well as several other members of the GATOR2 complex, have structural features consistent with coatomer proteins and membrane-tethering complexes. In line with the structural similarity to proteins that influence membrane curvature, we showed that three components of the GATOR2 complex, Mio, Seh1, and Sea2, localize to the outer surface of lysosomes, the site of TORC1 regulation. However, how GATOR2 inhibits GATOR1 activity, thus allowing for the robust activation of TORC1, remains unknown. Additionally, the role of the GATOR1 and GATOR2 complexes in both the development and physiology of multicellular animals remains poorly defined.
The GATOR1 complex regulates metabolic homeostasis and the response to nutrient stress in Drosophila.
We were interested in determining the role of the GATOR1 complex in the regulation of animal physiology. Towards this end, over the last year we characterized null mutants of the GATOR1 components nprl2, nprl3, and iml1 in Drosophila melanogaster. We found that all three mutants had inappropriately high baseline levels of TORC1 activity and reduced adult viability. Consistent with increased TORC1 activity, GATOR1 mutants exhibited a cell-autonomous rise in cell growth. Surprisingly, escaper nprl2 and nprl3 mutant adults had a profound motility defect. In line with a non-autonomous role in the regulation of systemic metabolism, expressing the Nprl3 protein in the fat body, a nutrient storage organ, and hemocytes but not muscles and neurons rescued the motility of nprl3 mutants. We also determined that nprl2 and nprl3 mutants fail to activate autophagy in response to amino acid limitation and are extremely sensitive to both amino acid and complete starvation. Thus, in Drosophila, in addition to maintaining baseline levels of TORC1 activity, the GATOR1 complex has retained a critical role in the response to nutrient stress. In summary, the TORC1 inhibitor GATOR1 contributes to multiple aspects of the development and physiology of Drosophila.
The GATOR2 component Wdr24 regulates TORC1 activity and lysosome function.
While the GATOR1 complex has been implicated in a wide array of human pathologies, including cancer and hereditary forms of epilepsy, the in vivo relevance of the GATOR2 complex remains poorly understood in metazoans. We defined the in vivo role of the GATOR2 component Wdr24 in Drosophila. Using a combination of genetic, biochemical, and cell-biological techniques, we demonstrated that Wdr24 has both TORC1–dependent and –independent functions in the regulation of cellular metabolism. Through the characterization of a null allele, we found that Wdr24 is a critical effector of the GATOR2 complex; it promotes the robust activation of TORC1 and cellular growth in a broad array of Drosophila tissues (Figure 1). Additionally, epistasis analysis between wdr24 and genes that encode components of the GATOR1 complex revealed that Wdr24 has a second critical function: the TORC1–independent regulation of lysosome dynamics and autophagic flux. Notably, we found that two additional members of the GATOR2 complex, Mio and Seh1, also play a TORC1–independent role in the regulation of lysosome function. These results represent a surprising and previously unrecognized function of GATOR2 complex components in the regulation of lysosomes. Consistent with our findings in Drosophila, through the characterization of a wdr24–/– knockout HeLa cell line, we determined that Wdr24 promotes lysosome acidification and autophagic flux in mammalian cells. Taken together, our data support the model wherein Wdr24 is a key effector of the GATOR2 complex, required for both TORC1 activation and the TORC1–independent regulation of lysosomes. Moreover, our data raise the interesting possibility that the GATOR2 complex regulates GATOR1 through the control of lysosome structure and/or function.
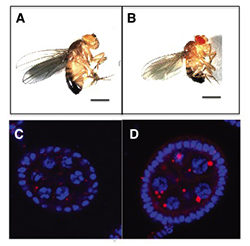
Click image to enlarge.
Figure 1. The GATOR2 component Wdr24 regulates growth and autophagy.
Representative images of (A) wild-type (WT) and (B) wdr241 mutant adult males. wdr241 males are notably smaller than WT males. Size bar is 100 μm. Representative images of (C) WT and (D) wdr241 egg chambers from well fed females stained with DAPI to mark DNA (blue) and LysoTracker (red) to mark autolysosomes. Note that wdr241 egg chambers accumulate large numbers of autolysosome-like structures under nutrient-replete conditions.
A conserved nutrient stress pathway regulates meiotic entry during oogenesis.
We defined a conserved pathway that controls the transition from the mitotic cycle to the meiotic cycle (Figure 2). In single-cell eukaryotes, the pathways that monitor nutrient availability are central to initiating the meiotic program and gametogenesis. A master regulator of metabolism in eukaryotes, TORC1 integrates information from several upstream signaling pathways. In Saccharomyces cerevisiae, an essential step in the transition to the meiotic cycle is the down-regulation of TORC1 by the GATOR1 complex in response to amino acid starvation. How metabolic inputs influence early meiotic progression and gametogenesis remains poorly understood in metazoans. We determined that, as is observed in yeast, the GATOR1 complex inhibits TORC1 activity to decelerate cellular metabolism and drive the mitotic/meiotic transition in developing ovarian cysts. In iml1 germline depletions, ovarian cysts undergo an extra mitotic division prior to meiotic entry. The TORC1 inhibitor Rapamycin can suppress this extra mitotic division. Thus, high TORC1 activity delays the mitotic/meiotic transition. Conversely, mutations in Tor, which encodes the catalytic subunit of the TORC1 complex, result in premature meiotic entry. Thus, the levels of TORC1 activity control the timing of the mitotic/meiotic decision in a metazoan. Taken together, our data indicate that the role of the GATOR1 complex in the regulation of meiotic entry has been conserved from single-cell to multicellular animals. The data strongly suggest that catabolic metabolism, triggered by low TORC1 activity, is a conserved feature of early meiosis in many eukaryotes.
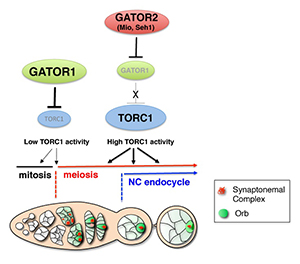
Click image to enlarge.
Figure 2. A model for the role of the GATOR1 and GATOR2 complexes during Drosophila oogenesis
The GATOR1 complex down-regulates TORC1 activity to promote the mitotic/meiotic transition after precisely four mitotic cyst divisions. Low TORC1 activity promotes the mitotic/meiotic transition. Whether the inhibition of TORC1 activity by the GATOR1 complex is required throughout the ovarian cyst divisions or precisely at the mitotic/meiotic juncture is currently undetermined and is thus presented as dashed arrows. After meiotic entry, the Mio and Seh1 components of the GATOR2 complex oppose the activity of GATOR1 complex, thus preventing the constitutive inhibition of TORC1 during later stages of oogenesis. High TORC1 activity is required to drive the nurse cell endoreplication cycle as well as for the maintenance of the meiotic cycle into later stages of oogenesis.
The GATOR1 complex promotes genomic stability during the early meiotic cycle.
Meiosis must accomplish two seemingly incompatible goals. First, it must faithfully copy and distribute genetic material to the next generation. Second, it must promote genomic diversity through meiotic recombination, a process initiated by the production of DNA double-stranded breaks (DSBs). In Drosophila females, entry into meiosis requires the down-regulation of TORC1 by the GATOR1 complex. However, why a nutrient sensor has a retained an essential role in the regulation of the early meiotic cycle in a metazoan remains unclear. We reasoned that, in addition to promoting meiotic entry, the GATOR1 complex might facilitate other events of the early meiotic cycle such as the highly conserved events of meiotic recombination. The controlled generation of DSBs initiates meiotic recombination in early prophase of meiosis I. Over the last year, we found that the GATOR1 complex promotes genomic stability in the presence of meiotic DSBs. nprl2– and nprl3–mutant oocytes exhibit a dramatic increase in the steady state number of DSBs (Figure 3). Moreover, in contrast to wild-type oocytes that repair their DSBs prior to oocyte growth, nprl2– and nprl3–mutant oocytes retain DSBs into late states of oogenesis. In nprl2– and nprl3–mutant ovarian cysts, the rise in unrepaired DSBs is accompanied by an increase in p53 activity, as monitored by a p53 reporter construct. p53 is a highly conserved transcription factor that mediates a response to genotoxic stress. Strikingly, inhibiting the generation of meiotic DSBs strongly suppressed p53 activity in the nprl31–mutant background. From these data, we conclude that the GATOR1 complex is required to maintain genomic stability in the presence of meiotic DSBs and is thus essential for oocytes to safely complete meiotic recombination. We also determined that nprl2 and nprl3 mutants exhibit profound encapsulation defects, with multiple ovarian cysts enclosed within single layer of follicle cells. Intriguingly, a similar encapsulation defect is observed in mutants of the Inducer of MEiosis 4 (ime4) gene in Drosophila. In budding yeast, IME4 is an essential regulator of early meiotic progression and gametogenesis. These data provide further support for the model that the GATOR1 complex has retained a critical role in the early meiotic cycle and early gametogenesis in metazoans.

Click image to enlarge.
Figure 3. nprl3–mutant ovarian cysts have elevated levels of DNA damage.
Note that WT ovarian cysts rapidly repair meiotic DSB, as indicated by the loss of gamma-H2Av in the early germarium. In contrast, nprl3 mutants retain high levels of gamma-H2Av into late stages of oogenesis.
Additional Funding
- NICHD Director's Challenge Award
Publications
- Wei Y, Reveal B, Cai, W, Lilly MA. The GATOR1 complex regulates metabolic homeostasis and the response to nutrient stress in Drosophila. G3 2016;6:3859-3867.
- Cai W, Wei Y, Jarnik M, Reich J, Lilly MA. The GATOR2 component Wdr24 regulates TORC1 activity and lysosome function. PLoS Genetics 2016;12(5):e1006036.
- Wei Y, Reveal B, Reich J, Laursen W, Senger S, Akar T, Iida-Jones I, Cai W, Jarnik M, Lilly MA. TORC1 regulators Iml1/GATOR1 and GATOR2 control meiotic entry and oocyte development in Drosophila. Proc Natl Acad Sci USA 2014;111:E5670-E5677.
- Wei Y, Lilly MA. The TORC1 inhibitors Nprl2 and Nprl3 mediate an adaptive response to amino-acid starvation in Drosophila. Cell Death Differ 2014;21:1460-1468.
Collaborators
- Juan Bonifacino, PhD, Section on Intracellular Protein Trafficking, NICHD, Bethesda, MD
- Brian Calvi, PhD, Indiana University, Bloomington, IN
- Chi-Hon Lee, MD, PhD, Section on Neuronal Connectivity, NICHD, Bethesda, MD
Contact
For more information, email mlilly@helix.nih.gov or visit http://cbmp.nichd.nih.gov/uccr.