Building the Zebrafish Lateral Line System
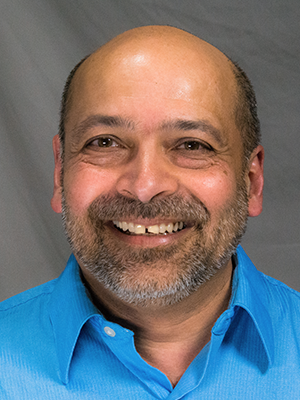
- Ajay Chitnis, MBBS, PhD, Head, Section on Neural Developmental Dynamics
- Damian E. Dalle Nogare, PhD, Staff Scientist
- Gregory Palardy, BS, Research Technician
- Chongmin Wang, MS, Research Technician
- Pritesh Krishnakumar, PhD, Postdoctoral Fellow
- Julia Boehling, BS, Postbaccalaureate Intramural Research Training Award Fellow
- Maryam Bolouri, BS, Postbaccalaureate Intramural Research Training Award Fellow
- Rachel Dansereau, BS, Postbaccalaureate Intramural Research Training Award Fellow
- Michael Hilzendeger, BS, Postbaccalaureate Intramural Research Training Award Fellow
- Megan Schupp, BS, Postbaccalaureate Intramural Research Training Award Fellow
Cells divide, move, adhere, and interact with their neighbors and their environment to determine the formation of multicellular organ systems with unique fates, morphologies, function, and behavior. Our goal is to understand how such interactions determine the self-organization of cell communities in the nervous system of the zebrafish embryo. The lateral line is a mechano-sensory system that helps sense the pattern of water flow over the fish and amphibian body; it consists of sensory organs called neuromasts, which are distributed in a stereotypic pattern over the body surface. Each neuromast has sensory hair cells at its center, surrounded by support cells that serve as progenitors for the production of more hair cells during growth and for the regeneration of neuromasts. The development of this superficial sensory system in zebrafish is spearheaded by the posterior Lateral Line primordia (pLLp), groups of about 150 cells formed on either side of a day-old embryo near the ear. Cells in the primordia migrate collectively under the skin to the tip of the tail, as they divide and reorganize to form nascent neuromasts, which are deposited sequentially from the lateral line's trailing end. Their journey is easily observed in live transgenic embryos with fluorescent primordium cells. Furthermore, a range of genetic and cellular manipulations can be used to investigate gene function and morphogenesis in the system. Understanding the self-organization of this relatively simple and accessible system in zebrafish will help elucidate the broader principles that determine cell-fate specification, morphogenesis, and collective cell migration in the developing vertebrate nervous system.
Signaling and mechanics interact to determine the number and size of protoneuromasts in the migrating primordium.
The zebrafish pLLp migrates under the skin, periodically forming and depositing neuromasts. Neuromasts are formed sequentially within the migrating primordium, starting from its trailing end as clusters of cells apically constrict and form epithelial rosettes. Periodic formation of such protoneuromasts is coordinated by interactions between the Wnt and Fgf signaling pathways. Wnt activity dominates in a leading domain, where it inhibits Fgf signaling and the formation of epithelial rosettes, while periodic formation of Fgf signaling centers in the wake of a shrinking Wnt system determines sequential reorganization of clusters of cells to form epithelial rosettes. While Fgf signaling may indeed seed the reorganization of cells to form epithelial rosettes, their precise number and the spacing of epithelial rosettes appears ultimately to be determined by a balance of forces promoting and opposing aggregation of cells to form these rosettes. Migration of cells in the leading Wnt–active domain is determined by chemokine signaling, while migration of trailing cells is determined by Fgf signaling. When chemokine-dependent migration is compromised, trailing cells, whose migration is dependent on Fgf signaling, keep moving, while leading cells, whose movement is dependent on chemokine signaling, slowdown. This results in local concertina-like buckling or compaction of the primordium and the fusion of trailing epithelial rosettes with leading rosettes to form fewer larger rosettes. However, such fusion is not observed when Fgf signaling, responsible for migration of trailing cells, is inhibited to slow primordium migration. These observations can be accounted for by a simple mechanics-based model of the migrating primordium, where local adhesive interactions promote aggregation, while tension along the length of the primordium, which pulls cells apart and is determined by the relative efficacy of leading chemokine-dependent and trailing Fgf–dependent collective cell migration, opposes such aggregation. In this manner, the migrating primordium provides a simple platform to visualize and understand how the interplay between signaling and mechanics determines emergent patterns of morphogenesis during development.
How does the dynamic behavior of individual cells determine tissue scale behavior in the migrating primordium?
Individual cells interact with their neighbors, change shape, and move to determine collective migration of the pLLp as protoneuromasts are periodically formed and deposited. In the past, we examined how various signaling systems, including the Wnt, Fgf, Notch, BMP, and chemokine pathways, affect morphogenesis of epithelial rosettes and specification of a central sensory hair-cell progenitor during the sequential formation of protoneuromasts in the migrating primordium, the collective migration of cells in the primordium, and the pace at which protoneuromasts are periodically formed and deposited by the migrating primordium. However, as it is technically difficult to examine the dynamic behavior of individual cells, our understanding has typically been limited to understanding these events at the tissue scale. However, in 2020, when physical work in the laboratory initially halted and then resumed at a much slower pace, Damian Dalle Nogare used the opportunity to develop a python-based pipeline to segment individual cells from high-resolution time-lapse imaging data sets obtained with DiSPIM (Dualview Inverted Selective Plane Illumination Microscope) imaging. The pipeline has now been used to segment and track all the cells in a migrating primordium. Using these data, we can reconstruct in three dimensions and over time the entire complement of cells in the pLLp and quantify and characterize various parameters associated with the diversity of cells in the primordium. We can also analyze the way in which cells interact during migration and morphogenesis of this tissue. These data have allowed us to confirm a prediction of our previous work, that superficial cells of the pLLp behave in a more fluid manner than the underlying rosette cells, undergoing more neighbor rearrangements than the more static neuromast-forming cells. Going forward, the pipeline will allow us to analyze with high precision the effect of manipulations that interfere with the normal development of the primordium, such as mutants and chemical inhibitors of signaling.
Additional Funding
- DIR Director’s Award FY21–22
- Developing Talent Award to Rachel Dansereau 2020–2022
Publications
- Dalle Nogare DE, Natesh N, Vishwasrao HD, Shroff H, Chitnis AB. Zebrafish Posterior Lateral Line primordium migration requires interactions between a superficial sheath of motile cells and the skin. eLife 2020;9:e58251.
Collaborators
- Hari Shroff, PhD, Laboratory of High Resolution Optical Imaging, NIBIB, Bethesda, MD
Contact
For more information, email chitnisa@mail.nih.gov or visit https://chitnislab.nichd.nih.gov.