Modeling the Biophysics of the Membrane
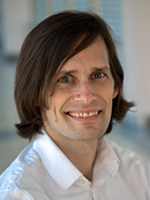
- Alexander J. Sodt, PhD, Head, Unit on Membrane Chemical Physics
- Andrew Beaven, PhD, Postdoctoral Fellow
- Amirali Hossein, PhD, Postdoctoral Fellow
- Henry H. Lessen, PhD, Postdoctoral Fellow
- Kayla Sapp, PhD, Postdoctoral Fellow
- Victor Chernomordik, PhD, Volunteer
- Kaitlyn Kerr, Summer Intern
- Onajia Stubblefield, Summer Intern
- Maya Wilson, Summer Intern
The integrity of lipid membranes is essential for life. They provide spatial separation of the chemical contents of the cell and thus make possible the electrical and chemical potential differences that are used to transmit signals and perform work. However, the membrane must be broken frequently to form, for example, new membrane structures in the cell. The simplest structure is a vesicle to transport cargo. Such vesicles are constantly cycled between organelles and the outer plasma membrane. Thus, there is a careful balance between boundary-establishing membrane fidelity and the necessary ability of the cell to change these boundaries.
The challenge in studying the membrane is its complexity. The membrane is a thin sheet of small molecules, i.e., lipids. There are hundreds of types of lipids in the cell. Each lipid changes the properties of the membrane in its vicinity, sometimes making the sheet stiffer, sometimes softer, and sometimes acting to bend the membrane into a ball or tube. Furthermore, the lipids are constantly jostling and tangling with both each other and with proteins embedded in the membrane. To predict of how membranes are reshaped thus requires not only knowing how lipids affect the properties of the membrane surface, but also the location of specific lipids.
The question as to how molecular scale features influence extensive biological processes must be answered in the language of physical laws. Physics is the language of mechanism at the molecular scale. The challenge is linking physics to the ‘big’ processes that happen in life. Our lab uses detailed physics-driven molecular simulation to ‘build up’ models that can be applied at the much larger level of the cell, which requires retaining important information and eliminating irrelevant details. The software our lab develops is based on the models that we are building. Thus, a broad objective of our research is to create a publicly available software package that can be used either as a stand-alone application for analyzing membrane-reshaping processes or as a library for cellular-scale modeling packages for which the role of the membrane may be unclear or unanticipated.
Another key component of our research is to seek the best possible validation of our models. Few techniques are able to yield molecular information about lipids. Recent breakthroughs that break the diffraction-limit barrier are typically only applicable to static structures much larger than a molecular dye. In contrast, lipids are small and dynamic. Our group is making a sustained effort to validate our simulation findings by applying neutron scattering techniques. This year, our lab initiated new collaborations on the basis of our previous years' work developing methodology for predicting the scattering signal from our simulations.
The projects use the NIH computing resources, including the Biowulf cluster, to run simulations and models. We use molecular dynamics software (such as NAMD and CHARMM) to conduct molecular simulations. In-house software development for public distribution is a key element of the lab's work.
New methodology probes the energy of complex lipid structures related to membrane fusion.
The work required to form a vesicle from the surface of the cell or an organelle is challenging to predict. Yet a model of this process is essential to understand many biological processes, including intracellular traffic and viral entry and exit.
A lipid bilayer is somewhere between an elastic sheet and the surface of a puddle. Like specks of dust on the surface of a puddle, lipids and proteins move along the surface. Yet like a sheet of rubber, the surface itself resists folding. It is not clear whether forming a vesicle from a sheet of lipids is more like covering a ball with a flat rubber sheet (impossible without cutting the sheet) or covering the ball with water (easy).
In the simulations described in our recent paper [Reference 1], we use advanced molecular simulation techniques to detect whether particular lipids are more stable composing vesicles on tubes, on sheets, or on exotic structures more closely resembling large cellular organelles. The study opens a path to determining how the chemistry of the hundreds of lipids in the cell determines the possible shapes biological machinery can create.
Separating individual lipids' contributions to membrane reshaping events
Membrane biophysicists have tested and modeled the effect of lipid chemistry on membrane shapes for decades. Studies have identified how specific lipids localize to high curvature and support membrane fusion and fission. Underlying these studies have been conflicting assumptions about how individual lipids act. Do they work locally, rearranging on the nanometer scale to cooperate with the cellular machinery that reshapes membranes? Or do they work globally, determining only whether the entire membrane is stiff or soft?
In our recently published study [Reference 2], we described the development of a novel theoretical analysis of how lipids shift and move on an undulating membrane, which we simulated on the high-performance computing resources at the NIH. Two results emerged: first, as determined by head-group chemistry, lipids act locally, allowing them to support very small, highly curved structures like membrane pores; second, lipids with saturated (well ordered) tail chemistry tended to prefer curving with a particular wavelength. This was unexpected and is a plausible mechanism for the interesting shapes and patterns that form from complex lipid mixtures.
The results of the study represent a significant opportunity to better understand how lipid gradients inside the cell promote or disinhibit vesicle traffic, which depends on the work required to reshape membranes.
Publications
- Beaven AH, Arnarez C, Lyman E, Bennett WFD, Sodt AJ. Curvature energetics determined by alchemical simulation on four topologically distinct lipid phases. J Phys Chem B 2021;125:1815–1824.
- Sapp KC, Beaven AH, Sodt AJ. Spatial extent of a single lipid's influence on bilayer mechanics. Phys Rev E 2021;103:042413.
Collaborators
- Drew Bennett, PhD, Lawrence Livermore National Laboratory, Livermore, CA
- Edward Lyman, PhD, University of Delaware, Newark, DE
Contact
For more information, email alexander.sodt@nih.gov or visit https://sodtlab.nichd.nih.gov.