Mechanisms of Synapse Assembly and Homeostasis

- Mihaela Serpe, PhD, Head, Section on Cellular Communication
- Peter Nguyen, Biological Laboratory Technician
- Tae Hee Han, PhD, Staff Scientist
- Wen Chieh Hsieh, PhD, Visiting Fellow
- Tho Huu Nguyen, PhD, Visiting Fellow
- Rosario Vicidomini, PhD, Visiting Fellow
- Thomas Brody, PhD, Special Volunteer
The purpose of our research is to understand the mechanisms of synapse development and homeostasis. The chemical synapse is the fundamental nervous-system communication unit that connects neurons to one another and to non-neuronal cells, and it is designed to mediate rapid and efficient transmission of signals across the synaptic cleft. The transmission forms the basis for the biological computations that underlie and enable our complex behavior. Crucial to the function is the ability of a synapse to change its properties, so that it can optimize its activity and adapt to the status of the cells engaged in communication and/or to the larger network comprising them. Consequently, synapse development is a highly orchestrated process coordinated by intercellular communication between the pre- and postsynaptic compartments and by neuronal activity itself. Our long-term goal is to elucidate the molecular mechanisms that regulate formation of functional synapses during development and fine-tune them during plasticity and homeostasis. We focus on four key processes in synaptogenesis: (1) trafficking of components to the proper site; (2) organizing those components to build synaptic structures; (3) maturation of the synapse to optimize its activity; and (4) homeostatic mechanisms that restore synapse activity to a set point after perturbations. We address the molecular mechanisms underlying these processes using a comprehensive set of approaches that include genetics, biochemistry, molecular biology, super-resolution imaging, and electrophysiology recordings in live animals and in reconstituted systems.
Because of its many advantages, we study these events in a powerful genetics system, Drosophila melanogaster, and use the neuromuscular junction (NMJ) as a model for glutamatergic synapse development and function. The fact that individual NMJs can be reproducibly identified from animal to animal and are easily accessible for electrophysiological and optical analysis makes them uniquely suited for in vivo studies on synapse assembly, growth, and plasticity. In addition, the richness of genetic manipulations that can be performed in Drosophila permits independent control of individual synaptic components in distinct cellular compartments. Importantly, the fly NMJ relies entirely on kainate-type receptors, a family of ionotropic glutamate receptors that impact synaptic transmission and neuronal excitability in the mammalian central nervous system but remain poorly understood. The Drosophila NMJ can thus be used to analyze and model defects in the structural and physiological plasticity of glutamatergic synapses, which are associated with a variety of human pathologies, from learning and memory deficits to autism. Drosophila has long served as a source of insight into human genetics, development, and disease, and the basic discoveries of our laboratory makes in the fly are likely to serve our overarching goal of understanding how chemical synapses are assembled and sculpted during development and homeostasis.
Synapse assembly
The first step in the assembly of synapses involves recruitment of synaptic components at the proper site. Prior to the motor neuron arrival, the ionotropic glutamate receptors (iGluRs) form small, nascent clusters on the muscle, which are distributed in the vicinity of future synaptic sites. Neuron arrival at its target muscle triggers the formation of large synaptic iGluR aggregates and promotes expression of more iGluRs to permit synapse maturation and growth. The iGluR clusters interact with the local cytoskeleton and other synaptic structures to maintain local density, which involves solving two fundamental problems common to all chemical synapses: (1) trafficking the components to the proper site, and (2) organizing those components to build synaptic structures. Recent advances, particularly from vertebrate iGluR biology, reveal that the solution to these problems is absolutely dependent on the activity of a rich array of auxiliary subunits that associate with the receptors. These highly diverse transmembrane proteins associate with iGluRs at all stages of the receptor life-cycle and mediate the delivery of receptors to the cell surface, their distribution, synaptic recruitment, associations with various postsynaptic density (PSD) scaffolds, and, importantly, their channel properties. iGluRs assembled from different subunits have strikingly different biophysical properties, and their association with different auxiliary subunits increases such diversity even further. In flies as in humans, synapse strength and plasticity are determined by the interplay between different postsynaptic iGluRs subtypes. At the fly NMJ, the type-A and type-B iGluRs consist of four different subunits: either GluRIIA or GluRIIB, plus GluRIIC, GluRIID and GluRIIE. In addition, a presynaptic autoreceptor, which contains the KaiRID (kainate receptor ID) subunit, controls basal neurotransmission.
We previously discovered that an obligatory auxiliary protein, Neto, is absolutely required for iGluR clustering and NMJ functionality. Our investigations uncovered essential roles for Neto during synapse development and strongly support the notion that trafficking of both postsynaptic iGluR subtypes and the presynaptic KaiRID–containing autoreceptor, their synaptic recruitment and stabilization, and their function are tightly regulated by Neto. Our results further indicate that the fly Neto isoforms (α and β) directly engage iGluRs as well as other intracellular and extracellular proteins to selectively regulate the distribution of iGluR subtypes, the recruitment of postsynaptic proteins, and the organization of postsynaptic structures. Given that iGluRs gating properties control the distribution and trafficking of these receptors in vivo, Neto could influence the synaptic recruitment of iGluRs by simultaneously controlling several steps in receptor trafficking and clustering and/or receptor function.
Neto belongs to a family of highly conserved auxiliary proteins that share an ancestral role in the formation and modulation of glutamatergic synapses. Vertebrate Neto1 and Neto2 were shown to modulate the properties of selective iGluRs, in particular the kainate-type receptors. Neto1/Neto2 double-knockout mice have defects in long-term potentiation and learning and memory, but the underlying mechanisms are extremely difficult to study owing to the low abundance of the kainate receptor channels and the small currents they elicit. Phylogenetic analyses indicated that both postsynaptic and presynaptic glutamate receptor subunits at the Drosophila NMJ belong to the kainate sub-family. Therefore, the fly NMJ, an essential synapse, is probably the best genetic system in which to probe the repertoire of both kainate receptors and Neto-type functions. Furthermore, using live imaging, we showed that Neto clusters at nascent NMJs at the time when iGluRs begin to accumulate and cluster. Also, Neto and iGluRs depend on each other for trafficking and stabilization at synaptic sites. Thus, mutants lacking any essential, shared iGluR subunits or Neto are completely paralyzed and die as embryos, with the iGluRs scattered as small aggregates away from the neuronal arbor. Mutants with suboptimal levels of Neto may live to adulthood, but they exhibit a wide range of behavioral deficits throughout development because of structural and functional synaptic defects, including reduced synaptic receptors, increased levels of extra-junctional iGluRs, and a series of PSD deficits. Our studies demonstrate that Neto engages the iGluRs on the cell membrane and together traffic to synaptic sites, where they form clusters. By controlling the clustering and trafficking of functional iGluR complexes, Neto directly controls synapse assembly, organization and maintenance of PSDs, and synapse functionality.
Neto–mediated intracellular interactions sculpt postsynaptic iGluR fields.
Type-A and type-B receptor complexes differ in their trafficking to the synapses, subsynaptic localization, and synaptic responses; at synapses with both type-A and type-B receptors, the dose of GluRIIA vs. GluRIIB is a key determinant of quantal size (the response of the muscle to the spontaneous release of a single synaptic vesicle). Previous work from our lab and others established that the synaptic recruitment of GluRIIA requires several postsynaptic components, a postsynaptic contribution that complements Neto’s ability to retain iGluRs at synaptic sites via extracellular interactions, the ‘clustering capacity.’ We found that mutants with high iGluR ‘clustering capacity’ that lack the relevant postsynaptic interactions cannot stabilize synaptic type-A receptors and instead incorporate type-B. We propose that the type-B receptors are the ‘default receptors’ at synapses with adequate clustering capacity, while the type-A receptors require an extensive network for their synaptic stabilization.
Evidence in support of the model comes from our studies on Neto-β, the predominant Neto isoform at the larval NMJ. Our developmental studies indicate that Neto-β controls the synaptic recruitment of iGluRs and of other postsynaptic components, such as P21–activating kinase (PAK), an important PSD component previously implicated in the stabilization of type-A receptors at postsynaptic sites. The neto-βnull synapses have reduced iGluRs synaptic clusters (Figure 1), in particular the type-A subtype, which reflects the drastic reduction in Neto net levels. However, a neto-βshort allele, which truncates part of the cytoplasmic domain and produces a shorter Neto-β variant, shows increased accumulation of synaptic GluRIIB and much reduced GluRIIA compared with control synapses. Thus, a short Neto-β, that cannot recruit type-A stabilizers, clusters type-B receptors. The finding suggests that Neto-β uses its cytoplasmic domain as an organizing platform to sculpt postsynaptic composition.
Interestingly, loss of Neto-α has no detectable effect on the synaptic accumulation of iGluRs. Instead, 3D-SIM analysis captured the enlarged receptor fields, which appeared to fill the small neto-αnull boutons (Figure 1). Muscle overexpression of a neto-α transgene fully rescued the PSD size of neto-αnull synapses. Thus, Neto-α limits the size of the postsynaptic receptor fields but has no detectable role in the organization of presynaptic specializations.
Figure 1. Neto isoforms have distinct roles in PSD organization and NMJ function.
Upper panels. Structure illumination (3D-SIM) images of control, neto-βnull, and neto-αnull synaptic boutons labeled for Brp (red), a presynaptic scaffold, GluRIIC (green), a share postsynaptic iGluR subunit, and Neto (blue).
Lower panels. Quantification of mEJP amplitude and quantal content (QC) values normalized to control captures a robust presynaptic compensatory response at neto-βnull and GluRIIA–mutant NMJs. In contrast, neurotransmitter release is reduced at neto-αnull terminals (as indicated by reduced QC and no significant reduction of mEJP amplitude), and there is no compensatory response upon further removal of the GluRIIA subunit.
Neto-α controls basal neurotransmission and synapse homeostasis.
In flies as in vertebrates, neuronal activity induces input-specific changes in the synaptic strength; at the larval NMJ, the postsynaptic sensitivity is primarily modulated via synapse-specific recruitment of GluRIIA. Robust homeostatic mechanisms keep synapses within an appropriate dynamic range such that the evoked potentials measured in the muscle remain constant from embryo to third instar larvae; reduced postsynaptic sensitivities (i.e., reduced GluRIIA activity) trigger a compensatory increase in quantal content (QC), the number of vesicle released by the neuron, which is referred to as presynaptic homeostatic potentiation (PHP).
The drastic reduction in synaptic iGluRs (primarily GluRIIA) at neto-βnull NMJs causes reduced mini frequency and amplitudes (mEJP), but such NMJs have normal evoked potentials owing to increased QC. Neto-α accounts for less than 10% of the Neto synaptic pool. Nonetheless, neto-αnull NMJs have normal mini amplitudes, but reduced basal neurotransmission. Interestingly, neuronal (but not muscle) expression of a neto-α transgene rescues the basal neurotransmission, indicating that Neto-α functions in the presynaptic compartment to modulate basal neurotransmission. We examined the homeostatic responses at neto-αnull NMJs using well established paradigms, including chronic (developmental) and acute (pharmacological) induction of PHP, as well as compensatory decrease in QC (triggered by neuronal overexpression of vGlut). Loss of presynaptic Neto-α renders such NMJs unable to express PHP but has no effect on homeostatic depression. Specifically, removal of GluRIIA during development leads to reduced quantal size (mEJP) and triggers PHP (increased QC), a PHP response that is not detectable in neto-αnull;GluRIIA double mutants (Figure 1). Also, application of sub-blocking concentrations of philanthotoxin (PhTx), a polyamine toxin derived from wasp venom, to semi-intact larval preparations triggers a fast reduction in quantal size and an increase in QC, so that the basal neurotransmission recovers within minutes. PhTx reduces the quantal size at neto-αnull NMJs, but the basal neurotransmission never recovers.
Given that a presynaptic kainate receptor, KaiRID, has been implicated in the control of basal neurotransmission and the expression of PHP, we examined whether Neto-α modulates KaiRID synaptic distribution and function. We found that Neto-α controls neurotransmitter release in a KaiRID–dependent manner. Furthermore, Neto-α is both required and sufficient for the PHP response, which includes an expansion of the vesicle release machinery. Interestingly, neuronal expression of Neto-β cannot rescue neto-αnull PHP deficits because Neto-β cannot traffic to the synaptic terminals and instead remains restricted to the somato-dendritic compartment. In contrast, a Neto variant with no intracellular domains (ΔCTD) can reach the presynaptic terminal and rescue the basal neurotransmission defects of neto-αnull, but it cannot restore the PHP. Our studies demonstrate that the intracellular part of Neto-α functions as a bona fide effector of PHP. The low-abundant Neto-α appears to be recruited and/or stabilized at synapses by the presynaptic KaiRID. The finding challenges our current assumption that auxiliary subunits 'assist' iGluRs and provides an exquisite example of an auxiliary protein that performs a key synaptic function with assistance from iGluRs.
Genetic interactors of neto
Our previous studies demonstrated that Neto enables trafficking of iGluRs at synaptic locations and the formation of stable clusters. At the same time Neto recruits postsynaptic components and controls postsynaptic differentiation. As Neto does not contain any catalytic domain, Neto functions via binding to iGluRs and other interaction partners. To identify proteins that interact with Neto and control synapse assembly at the developing NMJ, we conducted a series of biochemical and genetic screens. To screen for neto genetic interactors, we took advantage of a neto hypomorph, neto109, with drastically reduced NMJ function and synaptic structures, which exhibits locomotor deficits and partial lethality. The allele expresses less than 10% of the normal levels of Neto and has impaired PSD structures with much diminished synaptic iGluRs. Given that 50% of neto109 hypomorphs die developmentally, further reduction of synaptogenic proteins in hemizygous animals should increase lethality. Using this rationale, we set up a synthetic lethality screen to identify proteins that interact genetically with Neto and control the development of NMJ. In a screen of 418 overlapping deficiencies on chromosomes 2 and 3 (Bloomington Df kit) and also candidate interactors, we found and confirmed, firstly, known NMJ modulators such as Glass bottom boat (Gbb, a BMP ligand with critical roles during NMJ development), secondly, known components of synapse homeostasis, such as KaiRID, the presynaptic autoreceptor subunit, but thirdly, we also uncovered new players at the NMJ, such as Tenectin (Tnc), an integrin ligand required for NMJ development. Additional candidates revealed by this screen are currently being tested and examined for their potential role in synapse development and homeostasis.
Synapse maturation and homeostasis
Transsynaptic signaling modulates synapse assembly but also synapse maturation and developmental plasticity. How these signals monitor synaptic strength and relay this information to pre- and postsynaptic compartments is one of the central problems in neuroscience. Several families of developmentally important signaling molecules, including Wnt, TGF-β, and neurotrophins, have been implicated in sculpting synaptic junctions. Understanding the underlying mechanisms has been hindered by the complexity of these signaling networks, which are transduced by a web of transcriptional programs and local functions that govern multiple processes during neural development. For example, BMP signaling is critical for NMJ growth and neurotransmitter release and has been implicated in synapse homeostasis via unknown mechanisms. BMP signals via (1) a canonical pathway, which activates transcriptional programs with distinct roles in the structural and functional development of the NMJ in response to accumulation of phosphorylated Smad (pMad, an intracellular transducer of the BMP pathway) in motor neuron nuclei; and (2) a noncanonical, Mad–independent pathway, which connects synaptic structures to microtubules to regulate synapse stability. Intriguingly, pMad also accumulates at synaptic locations, but the biological relevance of this phenomenon remained a mystery for over a decade.
In previous work we discovered that postsynaptic type-A iGluRs, in their active state, together with Neto, trigger accumulation of pMad at active zones, the site of neurotransmitter release. We found that synaptic pMad marks a novel, local BMP pathway, genetically distinguishable from all other known BMP signaling cascades. This novel pathway does not contribute to NMJ growth but instead appears to provide a positive feedback loop that modulates the postsynaptic distribution of type-A and type-B receptors as a function of synapse activity.
Type-A receptors are the first to arrive at a nascent synapse; they form the ‘core’ of the receptor field and are surrounded by type-B receptors. Incorporation of type-A receptors in stable synaptic complexes depends on channel activity and requires an extensive postsynaptic network. Our studies on the novel BMP signaling modality indicate that the synaptic stabilization of type-A receptors also requires transsynaptic complexes. First, postsynaptic type-A receptors, in their active form, trigger accumulation of presynaptic pMad at active zones; secondly, genetic manipulations that disrupt pMad accumulation at the active zones trigger a reduction of synaptic type-A receptors, creating a positive feedback loop that stabilizes synaptic type-A receptors as a function of their activity.
The question arises as to how postsynaptic glutamate receptors modulate presynaptic pMad and are, in turn, stabilized by it. Given that synaptic pMad depends on active type-A receptors, we favor a model whereby Neto, via its BMP–binding CUB domains, connects active postsynaptic type-A receptors with presynaptic BMP/BMPR complexes (Figure 2, step 1). Such transsynaptic complexes could offer a versatile means for relaying synapse activity status to the presynaptic neuron via fast conformational modifications. At the same time, the complexes function as ‘accumulation centers’ for stabilizing type-A receptors at nascent synapses (step 2). Dissociation of these local complexes terminates further incorporation of type-A receptors and allows for recruitment of type-B subtypes, which mark mature synapses (steps 3–4). Importantly, the local BMP/BMPR complexes share limiting components with the other BMP signaling pathways, which control NMJ growth and synaptic stability, suggesting that neurons may use the novel BMP pathway to monitor synapse activity and then coordinate NMJ growth with synapse maturation and stabilization.
Figure 2.
Model for type-A receptor synaptic stabilization via transsynaptic interactions with local BMP signaling complexes
Cellular diversity in the Drosophila third instar larval ventral cord revealed by single-cell transcriptomics
To learn how motor neurons respond to different BMP signaling modalities or compensate for various postsynaptic perturbations, we performed single-cell RNA sequencing (scRNA-Seq) of larval ventral nerve cords (VNCs), the fly equivalent of the mammalian spinal cord. However, motor neurons represent a relatively small fraction (less than 2%) of the cells within the larval VNC. Without a larval VNC atlas available, it was practically impossible to recognize the motor neuron–specific transcriptomes and to begin comparing them under different conditions/genotypes. First, we had to assemble a larval VNC atlas that captures and characterizes the cellular diversity within the Drosophila third instar larvae. To accomplish this goal, we adapted and developed new protocols for dissociating single cells from fly larvae, and then assembled a custom multistage analysis pipeline that integrates modules contained in different R packages to ensure flexible, high-quality RNA-Seq data analysis. The work was conducted in collaboration with Steve Coon and James Iben.
We dissected third instar larvae VNCs, dissociated the cells, and sequenced about 31,000 high-quality single cells. Using un-supervised clustering algorithms, we clustered the cells into distinct populations. We then assigned the populations to specific cell types using known markers. Through a series of reiterative processes, we first identified eight different glia subtypes, each with distinct metabolic pathways. Secondly, based on the expression of neuroblast genes and the temporal determinant genes, we revealed a developmental trajectory leading from neural precursors to newborn neurons. We also detected novel differentially expressed genes along this trajectory. Thirdly, we identified over 40 types of clearly differentiated interneurons, each expressing unique combinations of transcription factors and neurotransmitters (VGlut, Gad1, VAChT, DAT). We found that most interneuron subtypes express multiple GPCRs (G protein–coupled receptors) and cell-recognition molecules, suggesting that many sensory modalities converge onto single cells to elicit specific motor functions/behaviors. We also identified a large motor neuron (MN) cluster (Figure 3), in which all cells express VGlut (encoding a glutamate transporter) but not the other neurotransmitter transporters or markers (Gad1, VAChT, DAT) (Figure 3). As expected, the MN transcriptomes are enriched in futsch (encoding a microtubule-binding protein involved in the formation of synaptic boutons at the neuromuscular junctions), proctolin (proc, encoding a neurohormone that modulates NMJ function through unknown mechanisms) and target of wit (twit, a BMP transcriptional target previously implicated in NMJ function).
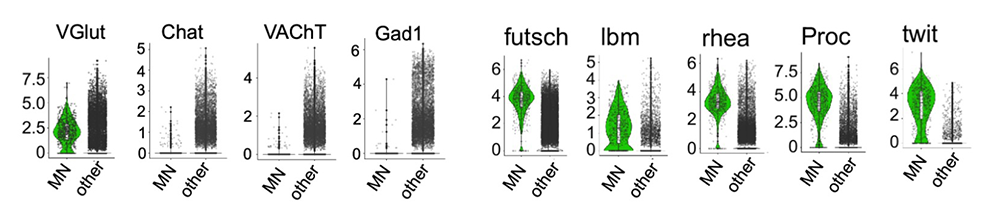
Click image to view.
Figure 3. Violin plots of transcripts enriched in larval glutamatergic motor neurons
The motor neuron (MN) cluster contains exclusively glutamatergic neurons, which express high levels of the vesicular glutamate transporter VGluT. The MNs do not express choline acetyltransferase (Chat), vesicular acetyl choline transporter (VAchT), or glutamic acid decarboxylase 1 (Gad1).
Additional MN–specific enriched transcripts include those of futsch (encoding a microtubule-binding protein involved in the formation of synaptic boutons at the neuromuscular junctions), lbm (tetraspanin implicated in axon guidance), rhea (or talin, essential for integrin function), Proc (neurohormone), and twit (target of BMP signaling pathway).
To increase our ability to examine the heterogeneity within the MN cluster, we marked the MNs with a twit-Gal4 promoter (and UAS-nls-GFP), dissociated VNCs from twit-GFP third instar larvae, and FACS–sorted the GFP–positive MNs. With this enrichment we were able to generate high quality scRNA-seq data for over 1,200 MNs. This reasonably large pool of MNs was then subdivided in 28 clusters. Several of the clusters are very well isolated and correspond to neurosecretory cells (expressing orcokinin, leukokonin or GPa), type II MNs (expressing Tdc2 and Vmat) and type III/peptidergic neurons (expressing the neurohormone bursicon, and the neuropeptide crustacean cardioactive peptide, CCAP). Our dataset reveals new markers for these types of neurons. For example, the AMPA–type glutamate receptor subunits GluRIA and GluRIB are primarily expressed in type II and type III neurons, suggesting that the modulatory activity of these neurons is regulated by glutamatergic input.
Drosophila has two type I MNs: tonic I-b neurons (with large synaptic boutons), which innervate single, dedicated muscles; and phasic I-s neurons, with small boutons and innervation spanning up to 7–8 muscles. Each larval hemisegment contains two I-s MNs, one projecting dorsally and one ventrally, and about 30 type I-b MNs, organized in distinct bundles that innervate subsets of body-wall muscles. Among the 28 MN clusters, two adjacent ones correspond to I-s MNs; they both express DIP-alpha (encoding a cell-surface molecule of the immunoglobulin superfamily), with the transcription factor gene eve marking the dorsally projecting I-s cluster. We found that anachronism (ana, encoding a secreted glycoprotein) specifically marks dorsally projecting I-s and confirmed this restricted expression using an ana-CRIMIC line. We also recognized different I-b MN bundles, projecting dorsally, ventrally, or laterally, such as the SNc bundle marked by the tey transcript (encoding a transcription factor). Interestingly, we found that all type I MNs show high CG11155 expression; CG11155 is predicted to code for a kainate-type glutamate receptor subunit. To search for the role of CG11155 during MNs development and function, we used CRISPR/Cas9 and RNAi to generate null mutants and tissue-specific knock downs. We found that CG11155 functions in MNs to ensure normal basal neurotransmission; in the absence of CG11155, basal neurotransmission is reduced to half that of control. This loss-of-function phenotype is reminiscent of the KaiRID loss-of-function defects. Given that iGluRs function as heterotetramers, usually dimers of dimers, our data suggest that KaiRID and CG11155 may be two subunits of the presynaptic autoreceptor that controls basal neurotransmission at the larval NMJ.
Our studies on the transcriptomes of larval MNs together with the assembly of a larval VNC atlas have already uncovered new molecules critical for synapse development and function and will provide a valuable resource for future studies on neuronal development and behavior.
Additional Funding
- NICHD Director’s Challenge Award Program in collaboration with Marry Dasso (NICHD) and Brian Oliver (NIDDK).
Publications
- Han TH, Vicidomini R, Ramos CI, Wang Q, Nguyen P, Jarnik M, Lee CH, Stawarski M, Hernandez RX, Macleod GT, Serpe M. Neto-alpha controls synapse organization and homeostasis at the Drosophila neuromuscular junction. Cell Rep 2020;32:107866.
- Nguyen TH, Han TH, Newfeld SJ, Serpe M. Selective disruption of synaptic BMP signaling by a Smad mutation adjacent to the highly conserved H2 helix. Genetics 2020;216:159–175.
- Nguyen TH, Vicidomini R, Choudhury S, Coon SL, Iben J, Brody T, Serpe M,. Single-cell RNA sequencing analysis of the Drosophila larval ventral cord. Curr Protoc 2021;e38.
- Vicidomini R, Nguyen TH, Choudhury S, Brody T, Serpe M. Assembly and exploration of a single cell atlas of the Drosophila larval ventral cord. Identification of rare cell types. Curr Protoc 2021;e37.
- Arnaoutov A, Lee H, Plevock Haase K, Aksenova V, Jarnik M, Oliver B, Serpe M, Dasso M. IRBIT directs differentiation of intestinal stem cell progeny to maintain tissue homeostasis. iScience 2020;23(3):100954.
Collaborators
- Steven Coon, PhD, Molecular Genetics Core, NICHD, Bethesda, MD
- Mary Dasso, PhD, Section on Cell Cycle Regulation, NICHD, Bethesda, MD
- James Iben, PhD, Molecular Genetics Core, NICHD, Bethesda, MD
- Gregory T. Macleod, PhD, Florida Atlantic University, Jupiter, FL
- Dragan Maric, PhD, Flow and Imaging Cytometry Core, NINDS, Bethesda, MD
- Mark Mayer, PhD, Scientist Emeritus, NINDS, Bethesda, MD
- Stuart Newfeld, PhD, School of Life Sciences, Arizona State University, Tempe, AZ
- Brian C. Oliver, PhD, Laboratory of Cellular and Developmental Biology, NIDDK, Bethesda, MD
Contact
For more information, email serpemih@mail.nih.gov or visit https://ucc.nichd.nih.gov.