Regulation of Childhood Growth
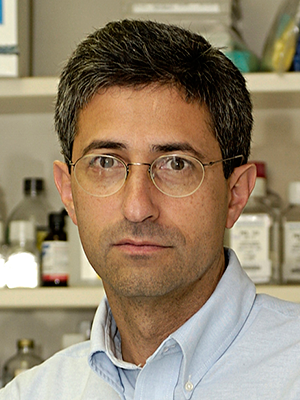
- Jeffrey Baron, MD, Head, Section on Growth and Development
- Kevin Barnes, PhD, Senior Research Assistant
- Julian Lui, PhD, Research Fellow
- Youn Hee Jee, MD, Senior Clinical Fellow
- Michal Ad, BS, Postbaccalaureate Fellow
- Yael Lebenthal, MD, Special Volunteer
- Jinhee Wang, PhD, Special Volunteer
- Quang Nguyen, BS, Special Volunteer
We investigate the cellular and molecular mechanisms governing childhood growth and development. We are especially interested in the mechanisms that allow rapid proliferation and hence rapid body growth in young mammals and subsequently suppress proliferation, thus setting a fundamental limit on the adult body size of the species. A goal of this work is to gain insight into the many human genetic disorders that cause childhood growth failure and overgrowth. Investigation of the identified growth-limiting mechanisms may lead to broader medical applications, because disruption of the mechanisms may contribute to oncogenesis, and conversely transient therapeutic suspension of growth-limiting mechanisms in adult cells might be used to achieve tissue regeneration.
Mechanisms limiting childhood growth
Body growth is rapid in infancy but subsequently slows and eventually ceases. The decline in growth rate during juvenile life occurs across mammalian species, including humans. In each species, growth slows concordantly in major organs to maintain body proportions. The growth deceleration results primarily from a decline in cell proliferation. We previously showed that the decline in cell proliferation is attributable in part to a growth-limiting genetic program that includes the postnatal down-regulation of many growth-promoting genes in multiple organs. We recently sought to explore the role of microRNAs (miRNAs) in the regulation of this growth-limiting genetic program (Reference 1).
We first performed microarray analysis to identify changes in miRNA expression during early postnatal life. Because miRNAs negatively regulate gene expression, we focused on miRNAs that were up-regulated with age and thus might contribute to the previously observed down-regulation of multiple mRNAs with age. We paid particular attention to miRNAs that were commonly up-regulated with age in multiple organs and thus more likely to contribute to the regulation of a genetic program that occurs simultaneously in each of these organs. The expression microarray analysis revealed that all members of the miR-29 family, miR-29a, miR-29-b, and miR-29-c, were strongly up-regulated with age in multiple organs in mice, findings that we confirmed by real-time PCR. Bioinformatic analysis showed that target sequences of the miR-29 family of microRNAs were overrepresented among genes that are down-regulated with age in multiple organs. We next focused on three predicted miR-29 target genes (Igf1, Imp1, and Mest), which are all growth-promoting. Using a luciferase-based assay and site-directed mutagenesis of the seed region, we found evidence that all three genes are indeed regulated by miR-29.
Taken together, the findings suggest that up-regulation of miR-29 during juvenile life helps orchestrate a juvenile multi-organ genetic program that involves the down-regulation of many growth-promoting genes with age. Therefore, the evidence supports the hypothesis that up-regulation of the miR-29 family of microRNAs serves as a regulatory mechanisms that allows rapid proliferation and body growth in early life but then suppresses proliferation, causing the rate of body growth to slow and eventually approach zero in adulthood.
Genes that control human linear growth
Children grow taller because their bones grow longer. Bone elongation occurs at the growth plate, a thin layer of cartilage found near the ends of juvenile bones. Consequently, many of the genes that control human height are expressed in and function in the growth plate. Based on this concept, we developed bioinformatic methods to identify genes that affect human height through local actions in the growth plate. The approach synthesizes data from our expression microarray studies of the growth plate, human disease databases, and a mouse knockout phenotype database. In collaboration with other research groups, we used the analytic approach to help identify genes that determine human height within loci detected by genome-wide association studies (Reference 2). In other collaborations, the method has been used to help identify human gene deletions that affect stature.
The work has important clinical implications because mutations in the genes that control growth lead to growth failure. Currently, for many children who experience linear growth failure, the etiology cannot be determined. Identifying new genes that control growth will likely allow us to determine the genetic cause of growth failure in many of these children.
Targeted treatment approaches for growth plate disorders
Mutations in hundreds of genes that are required for growth plate function give rise to disorders of skeletal growth, including the skeletal dysplasias, in which the bones are short and malformed, causing major disability. In addition to genetic disorders, acquired endocrine, nutritional, or inflammatory disorders can also impair bone growth at the growth plate, resulting in severe short stature.
Current treatment options for growth plate disorders are limited. Recombinant human growth hormone (GH) is used, but the efficacy is often suboptimal, particularly for children with skeletal dysplasias. Moreover, GH treatment carries the potential risks of increased intracranial pressure, slipped capital femoral epiphysis, insulin resistance, and promotion of malignancies. Because systemic administration of GH has limited efficacy and significant known and potential adverse effects, better treatments for growth plate disorders are needed.
Recent studies identified many paracrine factors that positively regulate growth plate chondrogenesis and therefore might be used therapeutically, including Indian hedgehog, bone-morphogenetic proteins, C-type natriuretic peptide, and WNT proteins. However, the development of these molecules into effective treatment has been hampered by their mechanism of action; such growth factors are produced locally and act locally in the growth plate, and thus do not lend themselves to systemic therapeutic approaches. We envisioned that these locally acting molecules could be targeted to the growth plate by linking them to cartilage-binding proteins, such as antibody fragments. When administered systemically, the hybrid molecules would be preferentially taken up by growth plate cartilage, and thus might greatly augment the therapeutic effect on the target organ while diminishing adverse effects owing to action on other tissues. Similarly, growth-promoting endocrine factors, such as GH and insulin-like growth factor-I, might be linked to cartilage-binding polypeptides and thereby targeted to the growth plate. Targeted endocrine therapy could potentially enhance the therapeutic effects on chondrogenesis and reduce effects on non-target tissues, thereby decreasing potential risks such as malignancy.
To develop cartilage-targeting therapy, we sought to identify polypeptides that home to cartilage tissue (Reference 3). In collaboration with scientists at the NCI, we employed a yeast display human antibody library and selected high-affinity binders to matrilin-3, an extracellular matrix protein expressed with high tissue specificity in cartilage. We identified antibody fragments that bind with high affinity to matrilin-3, as well as to cartilage tissue in vitro. In vivo, the antibody fragments homed specifically to cartilage tissue in mice (Figure).
Coupling the antibody fragments to endocrine and paracrine factors that stimulate chondrogenesis could be used to direct these potent molecules specifically to cartilage tissue and thus has the potential to open up new pharmacological approaches to treat childhood skeletal growth disorders.
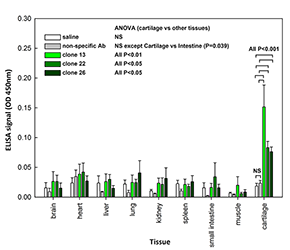
Click image to enlarge.
Homing of selected matrilin-3–binding antibody fragments to cartilage in vivo
Saline, non-specific antibody fragment, or purified antibody fragments 13, 22, and 26 were injected intravenously in 3-week-old mice. After 24 hours, various organs were collected and homogenized and tissue lysates used to coat plastic wells. The presence of antibody fragments was detected by ELISA with an anti-Fc antibody. All three antibody fragments were detected in cartilage, but not in non-cartilaginous organs. Saline and non-specific antibody served as negative controls. Data represent mean +/− SEM from five independent experiments (n=5).
Links between childhood growth and oncogenesis
When disrupted, many of the mechanisms that regulate mammalian body growth can contribute to the development of malignancies. We studied the role of two heparin-binding growth factors, pleiotrophin and midkine, in the regulation both of normal body growth and in the unregulated growth of malignancies. Recently, we measured midkine concentrations in fine-needle aspirate (FNA) samples from benign and malignant thyroid nodules to explore the possibility that midkine measurement might aid in the evaluation of thyroid nodules (Reference 4). Midkine was measured using a high-sensitivity sandwich ELISA and normalized to thyroglobulin concentration in the sample to adjust for tissue content in the aspirate. We found that, in FNA samples, the midkine/thyroglobulin ratio in papillary thyroid cancer was greater than in benign thyroid nodules, raising the possibility that the approach might provide adjunctive diagnostic or prognostic information to complement existing approaches.
Additional Funding
- Thrasher Research Fund Early Career Award to Dr. Youn Hee Jee (2014, ongoing): “The Role of Heparin Binding Growth Factors in Fetal and Childhood Growth”
- Clinical Center Genomic Opportunity Program (2104, ongoing): "Genetic Causes of Childhood Growth Failure"
- Merck-Serono Grant for Growth Innovation to Julian Lui (2014, ongoing): “Cartilage-Targeted Therapeutics for Growth Disorders”
- Endocrine Scholars Award in Growth Hormone Research to Dr. Julian Lui (2015, ongoing): “Cartilage-Targeted IGF-I for Treatment of Growth Disorders
Publications
- Kamran F, Andrade AC, Nella AA, Clokie SJ, Rezvani G, Nilsson O, Baron J, Lui JC. Evidence that upregulation of microRNA-29 contributes to postnatal body growth deceleration. Mol Endocrinol 2015; 29:921-932.
- Wood AR, Esko T, Yang J, et al. (GIANT Consortium). Defining the role of common variation in the genomic and biological architecture of adult human height. Nat Genet 2014; 46:1173-1186.
- Cheung CS, Zhu Z, Lui JC, Dimitrov D, Baron J. Human monoclonal antibody fragments targeting matrilin-3 in growth plate cartilage. Pharm Res 2015; 32:2439-2449.
- Jee YH, Celi FS, Kebebew E, Sampson M, Sacks DB, Remaley AT, Baron J. Midkine concentrations in fine-needle aspiration of benign and malignant thyroid nodules. Clin Endocrinol (Oxf) 2015; 83(6):977-84.
- Nilsson O, Weise M, Landman EB, Meyers JL, Barnes KM, Baron J. Evidence that estrogen hastens epiphyseal fusion and cessation of longitudinal bone growth by irreversibly depleting the number of resting zone progenitor cells in female rabbits. Endocrinology 2014; 155:2892-2899.
Collaborators
- Francesco Celi, MD, Clinical Endocrinology Branch, NIDDK, Bethesda, MD
- Weiping Chen, PhD, Genomics Core Facility, NIDDK, Bethesda, MD
- Andrew Dauber, MD, Boston Children’s Hospital, Boston, MA
- Dimiter Dimitrov, PhD, Laboratory of Experimental Immunology, Center for Cancer Research, NCI, Frederick, MD
- Joel Hirschhorn, MD, PhD, Harvard Medical School, Boston, MA
- Electron Kebebew, MD, Endocrine Oncology Branch, Center for Cancer Research, NCI, Bethesda, MD
- Ola Nilsson, MD, PhD, Karolinska Institute, Stockholm, Sweden
- Alan Remaley, MD, PhD, Cardiovascular and Pulmonary Branch, NHLBI, Bethesda, MD
- David B. Sacks, MB, ChB, FRCPath, Department of Laboratory Medicine, Clinical Center, NIH, Bethesda, MD
- James Segars, MD, Program in Reproductive and Adult Endocrinology, NICHD, Bethesda, MD
- Jan-Maarten Wit, MD, Universiteit Leiden, Leiden, The Netherlands
- Jack Yanovski, MD, PhD, Program in Developmental Endocrinology and Genetics, NICHD, Bethesda, MD
Contact
For more information, email jb233v@nih.gov or visit www.nichd.nih.gov/research/atNICHD/Investigators/baron.