Genetic and Genomic Studies in Normal Development and Diseases
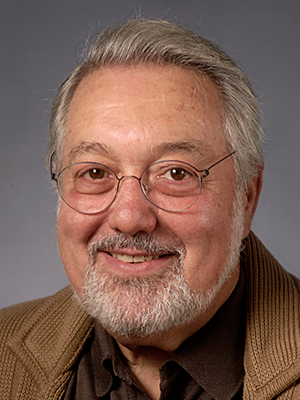
- Owen M. Rennert, MD, Head, Section on Clinical Genomics
- Margarita Raygada, PhD, CGC, Staff Clinician (Genetic Counselor)
Autism spectrum disorder (ASD) normally exhibits onset of symptoms before three years of age and is characterized by severe impairment in reciprocal socialization and communication skills and by repetitive or restrictive behaviors. It is a heterogeneous condition of multiple etiologies; no precise clinical assessment tools currently allow accurate definition of the many variants, nor are there biological markers to distinguish the variants. A rise in the number of children identified with ASDs, from five to 72 cases per 10,000 children in the USA and Europe, and the absence of definitive forms of therapy have given rise to mounting public concern. Our studies focus on the relationship between transcriptional networks formed during normal human brain development and genes that have been associated with ASDs. The work highlights the temporal and spatial (brain region) relationship between gene pathways and human brain development. Additional studies utilize neuronal cultures derived from induced pluripotent stem cells obtained from patients with ASD for studies on gene expression and neurophysiological properties.
The human diseases of premature aging in humans are characterized by early onset of aging phenotypes that are now known to be caused by mutations in various genes. Werner's syndrome (WS) is an adult progeroid syndrome caused by mutations in the Werner syndrome, RecQ helicase-like (WRN) gene. The WRN protein has been implicated in a variety of biochemical processes, including DNA replication, repair, recombination, telomere maintenance, and transcription. Loss of WRN results in genomic instability and dysfunctional telomeres. Skin fibroblasts from WS patients demonstrate reduced replication potential and accelerated senescence in culture, possibly a result of dysfunctional telomeres.
We also study the biological role of RNA–binding proteins in cell fate decision. The decision between self-renewal and differentiation determines the fate of pluripotent cells in the development of an organism. Our current knowledge of the cellular control and maintenance of pluripotency is derived mainly from studies on the action of specific transcription factors (for example, Oct4, Nanog, and Sox2) that control the expression of their downstream target genes. The availability, stability, and efficiency of translation of RNA transcripts of pluripotency-related genes represent further levels of regulation in the context of cell-fate decision. We are interested in the role of RNA–binding proteins in the maintenance of pluripotency. We use embryonic stem cells and embryonal carcinoma cells as model systems in which to study the biological functions of RNA–binding proteins (Lin28a and Pumilio/Nanos families) that are preferentially expressed in pluripotent cells. We are also interested in the regulatory mechanism that controls the cell type–specific expression patterns of these gene products.
It is apparent that, in addition to protein-coding transcripts, the mammalian genome expresses a large amount of non–protein-coding RNA transcripts. Among them, several long non–protein-coding RNAs (lncRNAs) and many microRNAs (miRNAs) have been found to regulate cellular physiology by tuning the expression of other genes at transcriptional and translational levels, respectively. In mammalian cells, alterations in the expression levels of individual protein-coding transcripts by epigenetic modifiers, which promote either histone acetylation or DNA demethylation, have been documented. However, the global change in the full transcriptome (comprising protein-coding RNA transcripts and their non–protein-coding counterparts) under the influence of such modifiers has never been analyzed systematically.
Autism research
Improved strategies for early identification of specific phenotypic characteristics of and biological markers (e.g., electrophysiological changes) for ASD would improve the effectiveness of treatment. The invasiveness of collecting primary neuronal tissue from patients could be circumvented by using induced pluripotent stem cells (iPSCs) that subsequently become neuronally differentiated. The successful reprogramming of human fibroblasts into an ES cell–like (iPSC) state in the Yamanaka laboratory (Takahashi et al., Cell 2007;131:861) and subsequent derivation of iPSCs from patients with ALS, Parkinson disease, and other disorders into cultured neural cells has served as the proof of principle. The breakthroughs made it possible for us to generate a cell-culture model of ASD by applying the technology for subsequent neural differentiation. We thus established fibroblast cultures from patients (subjects with autism) and non-affected controls; the cells were reprogrammed into an ES cell–like state. We clone the reprogrammed cell colonies, propagate them, and induce them to differentiate in vitro into neuronal cultures. Based on our underlying assumption that synaptic transmission is aberrant in autism, the patient-specific neuronal cultures are used for neuronal network analysis using the photo-conductive stimulation system described by Gutiérrez et al. (Eur J Neurosci 2009;30:2042). Briefly, spontaneous or pulse-stimulated activity of networks is measured by fluorescent optical techniques and the structural basis of the patterns analyzed by fractal dimension analysis. We have the capacity to characterize the arrangement and complexity of the networks' axonal architecture. We confirmed the methodology with hippocampal cultures of a rat model carrying the neurolignin mutation R471C-NL3 (identified in a subgroup of patients with ASD). We are attempting to evaluate membrane excitation and signal transduction in neural cells derived from patients with idiopathic autism.
We produced induced pluripotent cell lines (iPs) from four male children with idiopathic autism, four normal male siblings of these patients, four unaffected controls, and four patients with tuberous sclerosis; multiple clones of iP cultures from each group were established as well as neural progenitor cells and mature neural cultures from the cell lines. We developed a computer algorithm that quantitatively measures connectivity and synchronicity of activation. We are currently demonstrating proof of principle using neural cultures from hippocampal cultures derived from the mouse model of Rett syndrome. Studies are also under way using normal human neuronal cultures.
ASDs have a significant hereditary component, but the identified genetic loci are heterogeneous and complex. Consequently, there is a gap in our understanding of how diverse genomic aberrations can all result in the clinical ASD phenotype. Gene expression studies from autism brain tissue demonstrated aberrantly expressed protein-coding genes that may converge into common molecular pathways, potentially reconciling the strong heritability and shared clinical phenotypes with the disorder’s genomic heterogeneity. Regulation of gene expression is extremely complex and governed by many mechanisms, including noncoding RNAs. However, no study of ASD brain tissue has assessed changes in regulatory lncRNAs, which represent a large proportion of the human transcriptome and actively modulate mRNA expression. To determine whether aberrant expression of lncRNAs plays a role in the molecular pathogenesis of ASD, we profiled, by microarray, over 33,000 annotated lncRNAs and 30,000 mRNA transcripts from postmortem brain tissue of autistic and control prefrontal cortex and cerebellum. We detected over 200 differentially expressed lncRNAs in ASD, which were enriched for genomic regions containing genes related to neurodevelopment and psychiatric disease. Additionally, comparison of differences in expression of mRNAs between the prefrontal cortex and cerebellum within individual donors revealed that ASD brains had more transcriptional homogeneity. Moreover, this was also true of the lncRNA transcriptome (1, 2, 3, 4). Our results suggest that future investigation of lncRNA expression in the autistic brain may further elucidate the molecular pathogenesis of the disorder.
Premature aging syndromes
Previous studies on the pathogenesis of WS were limited to skin fibroblasts or virus-transformed lymphocytes. Animal models of the mutant WRN helicase cannot accurately recapitulate the WS phenotype observed in humans. Reprogramming of WS cells to iPSCs may provide a cell model for the study of the pathogenesis, especially for the differentiation of WS embryonic and adult stem cells into specific, affected cell types such as skin, bone, and muscle. To establish an iPSC model of WS, we reprogrammed patient fibroblasts with the transcriptional factors OCT4, SOX2, KLF4, and c-MYC. The efficiency of reprogramming for WS was much lower than for normal fibroblasts. Given that WS fibroblasts quickly reach senescence (in less than 20 population doublings), many cells failed to form colonies. To increase the efficiency of reprogramming, we tested several inhibitors, including vitamin C, the histone deacetylase (HDAC) inhibitor valproic acid, the MAP kinase inhibitor SB203580, and a cocktail of HDAC and TGF-β RI kinase inhibitors as well as small molecules reported by Sheng Ding’s group at the Gladstone Institute of Cardiovascular Disease, University of California San Francisco. Our preliminary data indicate that optimal efficiency was achieved with the HDAC and TGF-β RI kinase inhibitors; using these inhibitors, we observed the appearance of some colonies after 30 days of induction. The colonies could be expanded and expressed markers of embryonic stem cells (NANOG, OCT4, SOX2, SSEA3, SSEA4, TRA1-60, TRA1-81). By forming embryoid bodies in serum-containing medium, WS iPSCs could spontaneously differentiate into cells expressing markers of endoderm (α-fetoprotein), mesoderm (smooth muscle actin), and ectoderm (nestin).
Studies of transcription factors regulating cell renewal/proliferation
Epigenetic regulation of global transcriptome output
We have begun to examine the effect of epigenetic modifiers on transcriptome output in mammalian cells. Our preliminary analysis in several mouse cell lines indicated that the expression of specific lncRNAs is affected by the histone acetylation level. We are expanding the analysis to examine the effect of various epigenetic modifiers on full transcriptome output. From this study, we expect to identify subsets of non–protein-coding RNA transcripts that are commonly or distinctively regulated by different epigenetic modifiers, and we plan to investigate their roles in the homeostasis of the cells.
Publications
- Ziats MN, Grosvenor L, Rennert OM. Functional genomics of human brain development and implications for autism spectrum. Transl Psychiatry 2015; 5:e665.
- Mahfouz A, Ziats MN, Rennert OM, Lelieveldt BP, Reinders MJ. Shared pathways among autism candidate genes determined by co-expression network analysis of the developing human brain transcriptome. J Mol Neurosci 2015; 57(4):580-594.
- Ziats MN, Edmonson C, Rennert OM. The autistic brain in the context of normal neurodevelopment. Front Neuroanat 2015; 9:115.
- Rennert O, Lee TL. Epigenetics dynamics in development and disease. Int J Biochem Cell Biol 2015; 67:44.
- Tu J, Ng SH, Shui Luk AC, Liao J, Jiang X, Feng B, Lun Mak KK, Rennert OM, Chan WY, Lee TL. MicroRNA-29b/Tet1 regulatory axis epigenetically modulates mesendoderm differentiation in mouse embryonic stem cells. Nucleic Acids Res 2015; 43(16):7805-7822.
Collaborators
- Joan Han, MD, Program on Developmental Endocrinology and Genetics, NICHD, Bethesda, MD
- Dax Hoffman, PhD, Program in Developmental Neuroscience, NICHD, Bethesda, MD
- James Russell, DVM, PhD, Microscopy and Imaging Core Facility, NICHD, Bethesda, MD
- Susan Swedo, MD, PhD, Pediatrics and Developmental Neuroscience Branch, NIMH, Bethesda, MD
- Audrey Thurm, PhD, Pediatrics and Developmental Neuroscience Branch, NIMH, Bethesda, MD
Contact
For more information, email rennerto@mail.nih.gov.