Cell Cycle Regulation in Oogenesis
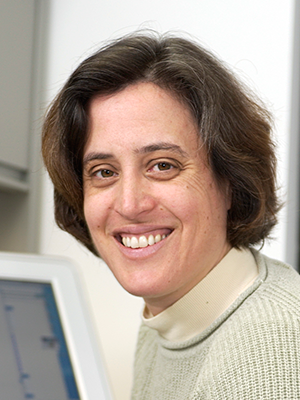
- Mary Lilly, PhD, Head, Section on Gamete Development
- Bradley Reveal, PhD, Postdoctoral Fellow
- Karine Fru, MD, Clinical Fellow
- Weili Cai, PhD, Visiting Fellow
- Youheng Wei, PhD, Visiting Fellow
- Kuikwon Kim, MS, Technician
- Megan Bannon, BS, Postbaccalaureate Fellow
- Nicholas Johnson, BS, Postbaccalaureate Fellow
The long-term goal of our laboratory is to understand how the cell-cycle events of meiosis are coordinated with the developmental events of gametogenesis. Chromosome mis-segregation during female meiosis is the leading cause of miscarriages and birth defects in humans. Recent evidence suggests that many meiotic errors occur downstream of defects in oocyte growth and/or the hormonal signaling pathways that drive differentiation of the oocyte. Thus, an understanding of how meiotic progression and gamete differentiation are coordinated during oogenesis is essential to studies in both reproductive biology and medicine. We use the genetically tractable model organism Drosophila melanogaster to examine how meiotic progression is instructed by the developmental and metabolic program of the egg.
In mammals, studies on the early stages of oogenesis face serious technical challenges in that entry into the meiotic cycle, meiotic recombination, and the initiation of the highly conserved prophase I arrest all occur during embryogenesis. By contrast, in Drosophila these critical events of early oogenesis all take place continuously within the adult female. Easy access to the early stages of oogenesis, coupled with available genetic and molecular genetic tools, makes Drosophila an excellent model for studies on meiotic progression and oocyte development.
To understand the regulatory inputs that control early meiotic progression, we are working to determine how the oocyte initiates and then maintains the meiotic cycle within the challenging environment of the ovarian cyst. Our studies focus on questions that are relevant to the development of all animal oocytes. What strategies does the oocyte use to protect itself against inappropriate DNA replication? How does the oocyte inhibit mitotic activity before meiotic maturation and the full growth and development of the egg? How does cell-cycle and metabolic status within the ovarian cyst influence the differentiation of the oocyte? To answer these questions, we have undertaken studies to determine the basic cell-cycle program of the developing ovarian cyst.
The SEA/GATOR complex: integrating developmental and metabolic signals in oogenesis
We are interested in how metabolism influences oocyte growth, development, and quality. Target of Rapamycin Complex 1 (TORC1) is a primary regulator of cell growth and metabolism, responding to multiple signals including nutrient availability, energy status, and growth factors. In the past year, we defined the role of the GATOR complex, a highly conserved upstream regulator of TORC1, in the regulation of meiotic progression, genomic stability, endomembrane dynamics, and oocyte development using Drosophila. We demonstrated that the GATOR1 complex down-regulates TORC1 activity to protect oocytes during times of amino acid scarcity and plays an essential role in the regulation of meiotic entry, genomic stability, and oocyte growth. We also found that the GATOR2 components Mio, Seh1, and Wdr24 oppose the activity of the GATOR1 complex in the female germline to promote growth in later stages of oogenesis. We thus conclude that the tight regulation of TORC1 activity by the GATOR1 and GATOR2 complexes is critical to oocyte development, meiotic progression, and genomic stability. Our data strongly suggest that the important role of nutrient stress pathways in the regulation of gametogenesis has been conserved from single-cell to multicellular animals.
In addition to our studies of oogenesis, we undertook a comprehensive characterization of the GATOR2 complex. We defined tissue-specific functions for several GATOR2 complex components. Surprisingly, our data support a dual role for the GATOR2 complex in the regulation of cellular metabolism and endomembrane dynamics.
The meiotic regulators Mio and Seh1 are components of the GATOR2 complex.
In earlier studies, we identified two genes, missing oocyte (mio) and seh1, that regulate meiotic progression and the maintenance of the oocyte fate. Both mio and seh1 are highly conserved from yeast to humans. In mio- and seh1-null mutants, oocytes enter the meiotic cycle and progress to pachytene. The meiotic cycle, however, is not maintained. Ultimately, a large fraction of mio and seh1 oocytes withdraw from meiosis, enter the endocycle, and become polyploid (Figure 1). Genetic and phenotypic analyses indicate that mio and seh1 act early in oogenesis, before the formation of the synaptonemal complex (SC) and meiotic recombination. Using biochemical strategies, we determined that Mio and Seh1 are components of a large multiprotein complex called the Seh1–associated (SEA) complex in yeast and the GATOR complex in higher eukaryotes. Recently, the SEA/GATOR complex was shown to be an upstream regulator of TORC1 activity. We found that TORC1 kinase activity is dramatically reduced in the ovaries of mio- and seh1-null mutants. Reduced TORC1 activity correlates with the strong reduction in growth observed in mio– and seh1– mutant ovaries. In contrast, mio and seh1 appear to have little effect on TORC1 activity and growth in most somatic tissues. Thus, there is a unique tissue-specific requirement for the TORC1 activators Mio and Seh1 in the female germline.
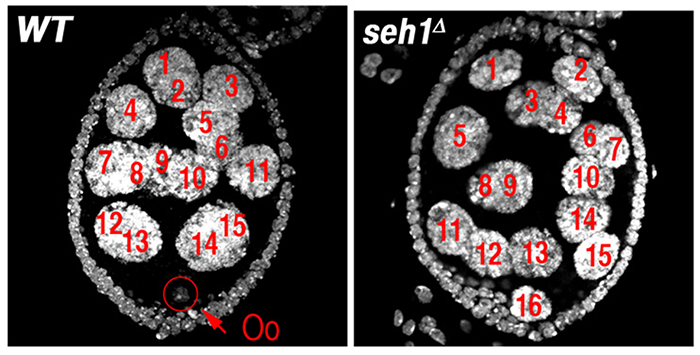
Figure 1. The GATOR1 component Seh1 is required for maintenance of the meiotic cycle.
Wild-type (WT) and seh1Δ egg chambers stained with the DNA dye DAPI. The seh1Δ egg chamber has 16 polyploid nurse cells but no oocyte (Oo).
The GATOR2 component Wdr24 uses TORC1–dependent and –independent pathways to regulate cellular metabolism.
To better define the in vivo role of the GATOR complex, we used whole-animal studies to dissect the function of the GATOR2 component Wdr24. Surprisingly, we found that Wdr24 regulates both TORC1–dependent and –independent processes. First, Wdr24 is a critical effector of the GATOR2 complex; Wdr24 promotes TORC1 activity and cellular growth in all tissues, which is in stark contrast to the GATOR2 components Mio and Seh1, which primarily function to promote TORC1 activity in the female germline. Second, Wdr24 is required for the TORC1–independent regulation of lysosome function and dynamics, representing a surprising, and previously unrecognized, role for the GATOR2 complex. Consistent with a role in the regulation of lysosomes, we showed that Wdr24 localizes to the surface of lysosomes and autolysosomes. Our results represent the first in vivo examination of the requirement for Wdr24 in a metazoan and provide a framework for future studies on the dual role of the GATOR2 complex in the regulation of cellular metabolism and endomembrane dynamics.
A conserved nutrient stress pathway regulates meiotic entry during oogenesis.
Over the past year, we defined a conserved pathway that controls the transition from the mitotic cycle to the meiotic cycle (Figure 2). In single-cell eukaryotes, the pathways that monitor nutrient availability are central to initiating the meiotic program and gametogenesis. A master regulator of metabolism in eukaryotes, TORC1 integrates information from multiple upstream signaling pathways. In Saccharomyces cerevisiae, an essential step in the transition to the meiotic cycle is the down-regulation of TORC1 by the Iml1/GATOR1 complex in response to amino acid starvation. How metabolic inputs influence early meiotic progression and gametogenesis remains poorly understood in metazoans. We demonstrated that, as is observed in yeast, the Iml1/GATOR1 complex inhibits TORC1 activity to slow cellular metabolism and drive the mitotic/meiotic transition in developing ovarian cysts. In iml1 germline depletions, ovarian cysts undergo an extra mitotic division prior to meiotic entry. The TORC1 inhibitor Rapamycin can suppress this extra mitotic division. Thus, high TORC1 activity delays the mitotic/meiotic transition. Conversely, mutations in Tor, which encodes the catalytic subunit of the TORC1 complex, result in premature meiotic entry. Thus, the levels of TORC1 activity control the timing of the mitotic/meiotic decision in a metazoan. Taken together, our data indicate that the role of the Iml1/GATOR1 complex in the regulation of meiotic entry has been conserved from single cell to multicellular animals. The data strongly suggest that catabolic metabolism, triggered by low TORC1 activity, is a conserved feature of early meiosis in many eukaryotes.
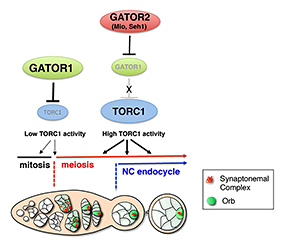
Click image to enlarge.
Figure 2. A model for the role of the GATOR1 and GATOR2 complexes during Drosophila oogenesis
The GATOR1 complex down-regulates TORC1 activity to promote the mitotic/meiotic transition after precisely four mitotic cyst divisions. Low TORC1 activity promotes the mitotic/meiotic transition. Whether the inhibition of TORC1 activity by the GATOR1 complex is required throughout the ovarian cyst divisions or precisely at the mitotic/meiotic juncture is currently undetermined and thus is presented as dashed arrows. After meiotic entry, the Mio and Seh1 components of the GATOR2 complex oppose the activity of GATOR1 complex, thus preventing the constitutive inhibition of TORC1 during later stages of oogenesis. High TORC1 activity is required to drive the nurse cell endoreplication cycle as well as for the maintenance of the meiotic cycle into later stages of oogenesis.
The GATOR1 components Nprl2 and Nprl3 mediate an adaptive response to amino acid starvation in Drosophila.
In yeast, the Nitrogen permease regulators 2 and 3 (Npr2 and Npr3) are components of the Iml1/GATOR1 complex; they mediate an essential response to amino acid limitation upstream of TORC1. In mammals, the Npr2 ortholog, Nprl2, encodes a putative tumor suppressor gene, which inhibits cell growth and enhances sensitivity to numerous anticancer drugs including cisplatin. However, the precise role of Nprl2 and Nprl3 in the regulation of metabolism in metazoans remains poorly defined. We determined that the central importance of Nprl2 and Nprl3 in the response to amino acid starvation has been conserved from single celled to multicellular animals. We found that, in Drosophila, Nprl2 and Nprl3 physically interact and are targeted to lysosomes and autolysosomes, the sites of TORC1 activation. Using oogenesis as a model system, we found that Nprl2 and Nprl3 inhibit TORC1 signaling in response to amino acid starvation. Moreover, the inhibition TORC1 by Nprl2/3 is critical to the preservation of female fertility during times of protein scarcity. In young egg chambers, the failure to down-regulate TORC1 in response to amino acid limitation triggers apoptosis (Figure 3). Thus, our data suggest the presence of a metabolic checkpoint that initiates a cell death program in the oocyte when, during periods of amino acid and/or nutrient scarcity, TORC1 activity remains inappropriately high.
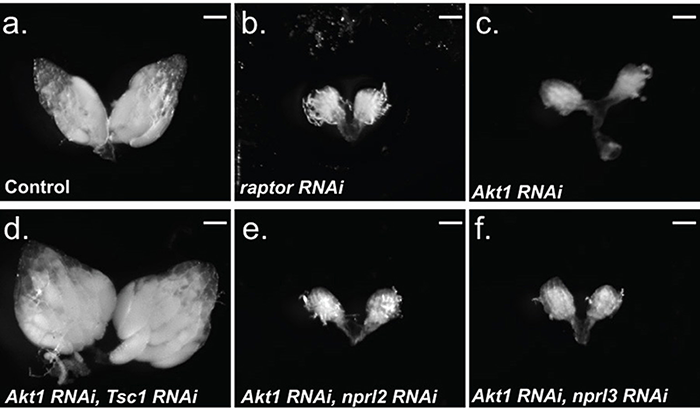
Figure 3. The TORC1 inhibitor Tsc1 is downstream of Akt1 in the female germline.
a. nanos-GAL4; UAS-mCherry RNAi, b. nanos-GAL4; UAS-raptor RNAi, c. nanos-GAL4; UAS-Akt1 RNAi, d. nanos-GAL4; UAS-Akt1 RNAi/UAS-Tsc1 RNAi, e. nanos-GAL4; UAS-Akt1 RNAi/UAS-nprl2 RNAi, and f. nanos-GAL4; UAS-Akt1 RNAi/UAS-nprl3 RNAi flies were cultured on standard fly media with wet yeast two days prior to dissection. The germline-specific driver nanos-GAL4 was used to drive expression of the RNAi constructs. (a, d) 100% of the ovaries examined contained mature (stage 13–14) egg chambers. (b, c, e, f) 0% of the ovaries examined contained egg chambers beyond stage 5. (a–f) N>50 ovaries. Note that, while knocking down Tsc1 in the female germline results in a block to oogenesis, the co-depletion of the TORC1 inhibitor Tsc1, the downstream target of Akt1, restores oogenesis. Thus, Tsc1 is epistatic to Akt1 in the female germline. Size bar 50 μm.
The TSC1/2 complex is a TORC1 inhibitor that is required to maintain baseline levels of TORC1 activity in most cell types. As observed with nprl2 and nprl3, we found that depleting Tsc1 in the female germline renders oogenesis acutely sensitive to amino acid stress. Yet the TSC1/2 complex is not activated by amino acid starvation. One possible model to explain these data is that, in the female germline, the TSC1/2 complex is required to maintain baseline levels of TORC1 activity while Nprl2/Nprl3 are required to specifically down-regulate TORC1 activity in response to amino acid scarcity. Therefore, both TORC1 inhibitor pathways are required to repress TORC1 activity in order to prevent oocytes from being shunted into the apoptotic pathway in response to amino acid scarcity (Figure 4). We predict that, in many tissues, the two independent TORC1–inhibitory pathways work in concert to fine-tune TORC1 activity in response to various developmental and environmental inputs. We also found that Tsc1 is a critical downstream effector of Akt1 in the female germline.
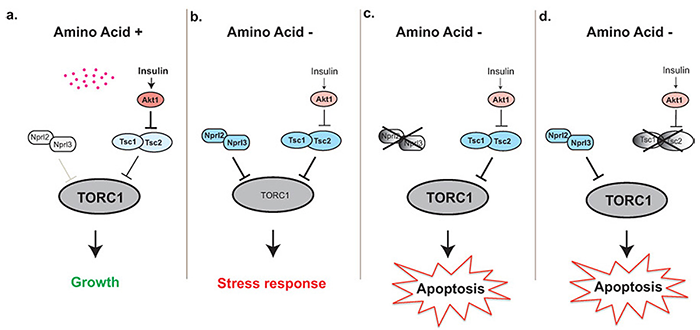
Figure 4. The TORC1 inhibitors Nprl2/3 and Tsc1/2 prevent apoptosis and protect young oocytes during amino acid starvation.
a. In the presence of amino acids, the TORC1 inhibitors Nprl2/3 are inactive while the insulin pathway inhibits the activity of Tsc1/2. Thus, TORC1 activity is high, driving anabolic metabolism and growth. b. In the absence of amino acids, Nprl2/3 functions to inhibit TORC1 activity. Amino acid starvation also results in reduced insulin signaling, leading to activation of the Tsc1/2 complex owing to the reduced activity of its inhibitor Akt1. Together, Nprl2/3 and Tsc1/2 sufficiently inhibit TORC1 activity to induce an essential stress response. c. In nprl2 or nprl3 knockdowns, cells fail to adequately down-regulate TORC1 activity in response to amino acid starvation, triggering apoptosis. d. In Tsc1 knockdowns, TORC1 activity also remains inappropriately high during amino acid starvation, triggering apoptosis.
Additional Funding
- NICHD Director's Challenge Award
Publications
- Wei Y, Reveal B, Reich J, Laursen W, Senger S, Akar T, Iida-Jones I, Cai W, Jarnik M, Lilly MA. TORC1 regulators Iml1/GATOR1 and GATOR2 control meiotic entry and oocyte development in Drosophila. Proc Natl Acad Sci USA 2014; 111:E5670-E5677.
- Wei Y, Lilly MA. The TORC1 inhibitors Nprl2 and Nprl3 mediate an adaptive response to amino-acid starvation in Drosophila. Cell Death Differ 2014; 21:1460-1468.
- Kassis JA, Lilly MA. PRC2 goes solo in the Drosophila female germline. Dev Cell 2013; 26:329-330.
Collaborators
- Juan Bonifacino, PhD, Cell Biology and Metabolism Program, NICHD, Bethesda, MD
- Brian Calvi, PhD, Indiana University, Bloomington, IN
- Chi-Hon Lee, MD, PhD, Program in Cellular Regulation and Metabolism, NICHD, Bethesda, MD
- Kim McKim, PhD, Waksman Institute of Microbiology, Rutgers, The State University of NJ, Piscataway, NJ
Contact
For more information, email mlilly@helix.nih.gov or visit http://cbmp.nichd.nih.gov/uccr.