Biophysics of Large Membrane Channels
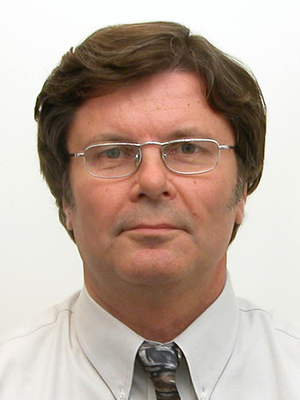
- Sergey M. Bezrukov, PhD, DSci, Head, Section on Molecular Transport
- Tatiana K. Rostovtseva, PhD, Staff Scientist
- Oscar Teijido Hermida, PhD, Visiting Fellow
- David P. Hoogerheide, PhD, Visiting Fellow, NIH/NIST Joint Sponsorship Program
We explore the physical mechanisms that are responsible for the functioning and regulation of mitochondrial and bacterial beta-barrel channels under normal and pathological conditions. These membrane proteins, which form large water-filled pores, are the gateways for metabolite exchange between various cellular compartments and cells. Because they are also recognized as multifunctional membrane receptors and components of many toxins, beta-barrel channels are also promising as novel drug targets. Our main strategy is to reconstitute the proteins into planar lipid membranes, which allows us to study channels at the single-molecule level by high-resolution recording of ionic currents. Combining physical theory of channel-facilitated transport with experiments on channel reconstitution, we seek to develop new approaches to the treatment of various diseases for which regulation of transport through ion channels plays a key role.
To understand the general principles involved in channel regulation at the molecular level, we work with a variety of proteins and channel-forming peptides. Among them are VDAC (the Voltage-Dependent Anion Channel from the outer membrane of mitochondria), alpha-Hemolysin (a toxin from Staphylococcus aureus), translocation pores of Bacillus anthracis (PA63), Clostridium botulinum (C2IIa) and Clostridium perfringens (Ib) binary toxins, Epsilon toxin (from Clostridium perfringens), OmpF (a general bacterial porin from Escherichia coli), LamB (a sugar-specific bacterial porin from Escherichia coli), OprF (porin from Pseudomonas aeruginosa), Syringomycin E (a lipopeptide toxin from Pseudomonas syringae), and the bacterial peptide TisB involved in persister cell biofilm formation. We also use Gramicidin A (a linear pentadecapeptide from Bacillus brevis) as a molecular sensor of membrane mechanical properties. To study the channel-forming proteins under precisely controlled conditions, we first isolate them from the host organisms, purify them, and then reconstitute them into planar lipid bilayers with precisely controlled physical properties. This allows us to explore channel interactions with the lipid membrane when modified by volatile anesthetics, with cytosolic proteins such as tubulin and alpha-synuclein, and with newly synthesized drugs such as blockers of the translocation pores of bacterial toxins. By learning the physics, chemistry, and physiology of channel functioning, we strive to determine how to design new agents and strategies that effectively correct the deviant interactions associated with disease.
Alpha-synuclein interaction with VDAC as a mechanism of mitochondrial regulation and toxicity in Parkinson disease
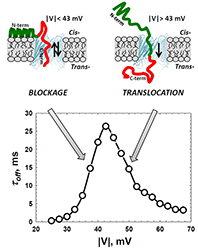
Click image to enlarge.
Figure 1. Alpha-synuclein interaction with VDAC
The cross-over in the voltage dependence of the residence time of alpha-synuclein in the VDAC pore separates regimes of reversible blockage and protein translocation through the pore. Depending on the amplitude of the applied voltage, it either reversibly blocks or translocates through the pore. At relatively low voltages, upon the capture by the channel, the field pulls on the negatively-charged C-terminus (red) with a force that is not enough to detach the N-terminal part (green) from the membrane surface. This results in a reversible capture of the C-terminus and in an exponential increase of its residence time in the pore with the voltage. Voltages above a certain threshold (around 45 mV) are high enough to induce detachment of the N-terminal part and to pull the whole peptide chain through the pore. In this translocation regime, the increasing field threads protein through the pore faster, which results in the lower residence time (Reference 1).
Emerging evidence establishes the critical role of mitochondria in the pathogenesis of neurodegenerative diseases such as Parkinson’s disease (PD) and Alzheimer’s disease. Dysfunction of mitochondrial enzyme complexes, impaired oxidative phosphorylation, increased production of reactive oxygen species, mitochondrial outer membrane permeabilization, enhanced apoptosis, and morphological alterations of mitochondria have been associated with these pathologies. Neurons are especially sensitive to mitochondrial dysfunction because of their high demand for energy and the characteristic subcellular distribution of their mitochondria. Participation of the small, intrinsically disordered protein alpha-synuclein (α-syn) in PD pathogenesis has been well documented. Although recent research demonstrated the involvement of α-syn in mitochondrial dysfunction in neurodegeneration and suggested direct interaction of α-syn with mitochondria, the molecular mechanism(s) of α-syn toxicity and its effect on neuronal mitochondria remain sketchy. Using the channel reconstitution technique, we found that, at nanomolar concentrations, α-syn reversibly blocks VDAC, the major transport pore of the mitochondrial outer membrane, a pore that controls most of the metabolite fluxes in and out of the mitochondria. It is clear that any restriction of metabolite exchange through VDAC may lead to disturbance of the mitochondrial energetic function and therefore to cell metabolism dysfunction. Moreover, through the detailed analysis of the blockage kinetics, we came to the conclusion that α-syn is able to translocate through the channel. Implication of VDAC in regulation of α-syn entrance into mitochondria may be of an immediate importance for the understanding of mitochondrial dysfunctions in PD. Indeed, after reaching the intermembrane space, α-syn may target complexes of the mitochondrial respiratory chain in the inner mitochondrial membrane. Thus, our results suggest that, depending on the physiological conditions, α-syn interaction with VDAC could be involved in regulation of normal mitochondrial respiration, in α-syn–induced mitochondrial dysfunction, or both. Supporting our in vitro experiments, a yeast model of PD showed that α-syn toxicity in yeast depends on VDAC, demonstrating α-syn interaction with VDAC in living cells. The functional interactions between VDAC and α-syn, revealed by our study, point toward the long sought-after physiological and pathophysiological roles for monomeric α-syn in PD and in other α-synucleinopathies and, for the first time, suggest a pathway for α-syn across the mitochondrial outer membrane. We believe that these results can reconcile several previous conflicting observations of various α-syn effects on mitochondrial bioenergetics.
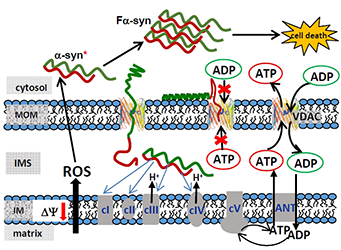
Click image to enlarge.
Figure 2. A model of mitochondrial outer membrane permeability regulation and α-syn–induced cytotoxicity
The cross-over in the voltage dependence of the residence time of alpha-synuclein in the VDAC pore separates regimes of reversible blockage and protein translocation through the pore. Depending on the amplitude of the applied voltage, it either reversibly blocks or translocates through the pore. At relatively low voltages, upon the capture by the channel, the field pulls on the negatively-charged C-terminus (red) with a force that is not enough to detach the N-terminal part (green) from the membrane surface. This results in a reversible capture of the C-terminus and in an exponential increase of its residence time in the pore with the voltage. Voltages above a certain threshold (around 45 mV) are high enough to induce detachment of the N-terminal part and to pull the whole peptide chain through the pore. In this translocation regime, the increasing field threads protein through the pore faster, which results in the lower residence time (Reference 1).
Complexity of voltage gating of beta-barrel channels
The model is based on alpha-synuclein (α-syn) interaction with and translocation through VDAC. VDAC blockage by α-syn disrupts ATP/ADP exchange between mitochondria and the cytosol, thus distorting the substrate balance for the adenine-nucleotide translocator (ANT) located in the inner membrane (IM). This leads to depletion of ATP-synthase (cV) with ADP, reduced mitochondrial potential, and impaired oxidative phosphorylation. By crossing the mitochondrial outer membrane (MOM) through VDAC into the intermembrane space (IMS), α-syn is able to directly target complexes of the electron transport chain (cI, cII, cIII, and cIV) in the IM. This could lead to mitochondrial dysfunction characterized by enhanced production of the reactive oxygen species (ROS). In turn, ROS induces monomeric α-syn oxidation in the cytosol, causing α-syn oligomerization and consequent amplification of fibrillar α-syn (Fa-syn) neurotoxicity, eventually resulting in cell death (Reference 1).
Physical theory of transport
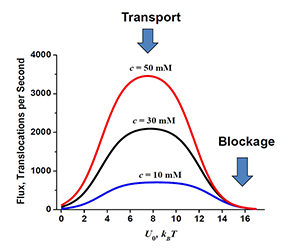
Click image to enlarge.
Figure 3. Optimal transport versus channel blockage
The flux through the channel depends on the strength of channel-particle interactions in a non-monotonic way. For the cylindrical channel geometry of 5 nm length and 0.2 nm radius and the range of the particle concentrations specified in the figure, the depth of the rectangular well that optimizes the transport is around 6 to 10 kBT per molecule. Blockage of the channel, which is often a mechanism of channel regulation in nature, is achieved at higher well depths (Reference 5).
This year, we focused on the problems of (1) bulk-mediated surface diffusion and (2) biased diffusion in three-dimensional comb-like structures. Bulk-mediated surface diffusion is attracting much attention in both theory and experiment owing to its well recognized relevance to a wide variety of processes in nature and technology. This kind of diffusion characterizes the motion of particles on a surface that is in a contact with the bulk in a way that allows for the dynamic partitioning of the particles between the surface-bound and bulk states. As a result, particles intermittently diffuse on the surface and in the bulk, allowing for an accelerated propagation of the particles over the surface when bulk diffusivity is significantly larger than its surface counterpart. We were motivated by our recent studies of the interaction between certain cytosolic proteins and their membrane-embedded targets, which demonstrated that it involves bulk-mediated diffusion over the membrane surface as an essential step. We developed a new approach to the problem, which reduces its solution to that of a two-state problem of the particle transitions between the surface and the bulk layer, focusing on the cumulative residence times spent by the particle in the two states. The times are random variables, the sum of which is equal to the total observation time. The advantage of the approach is that it allows for a simple exact analytical solution for the double Laplace transform of the conditional probability density of the cumulative residence time spent on the surface by the particle for the observation time. The solution was used to find the Laplace transform of the particle mean square displacement and to analyze the peculiarities of its time behavior over the entire range of time. In the case of biased diffusion in three-dimensional comb-like structures, we developed a formalism that allowed us to derive analytical expressions for the Laplace transforms of the first two moments of the particle displacement along the main tube axis. Through these one can find the time dependencies of the two moments for arbitrary values of both the drift velocity and the dead-end length, including the limiting case of infinitely long dead ends, where the unbiased diffusion becomes anomalous at sufficiently long times. The results give us a set of rigorous tools for quantitative analysis of experimental findings.
Additional Funding
- NRC grant “Developing a bio-mimetic activating platform for the mitochondrial outer membrane” from NIH(NIBIB)/NIST Joint Sponsorship Program (2013-2015).
Publications
- Rostovtseva TK, Gurnev PA, Protchenko O, Hoogerheide DP, Yap TL, Philpott CC, Lee JC, Bezrukov SM. Alpha-synuclein shows high-affinity interaction with voltage-dependent anion channel suggesting mechanisms of mitochondrial regulation and toxicity in Parkinson disease. J Biol Chem 2015; 290:18467-18477.
- Rappaport SM, Teijido O, Hoogerheide DP, Rostovtseva TK, Berezhkovskii AM, Bezrukov SM. Conductance hysteresis in the voltage-dependent anion channel. Eur Biophys J 2015; 44:465-472.
- Berezhkovskii AM, Dagdug L, Bezrukov SM. Biased diffusion in three-dimensional comb-like structures. J Chem Phys 2015; 142:134101.
- Berezhkovskii AM, Dagdug L, Bezrukov SM. A new approach to the problem of bulk-mediated surface diffusion. J Chem Phys 2015; 143:084103.
- Berezhkovskii AM, Bezrukov SM. On the applicability of entropy potentials in transport problems. Eur Phys J Spec Top 2014; 223:3063-3077.
Collaborators
- Vicente M. Aguilella, PhD, Universidad Jaume I, Castellón, Spain
- Alexander M. Berezhkovskii, PhD, Division of Computational Bioscience, CIT, NIH, Bethesda, MD
- Susan K. Buchanan, PhD, Laboratory of Molecular Biology, NIDDK, Bethesda, MD
- Leonid V. Chernomordik, PhD, Program in Physical Biology, NICHD, Bethesda, MD
- Leonardo Dagdug, PhD, Universidad Autónoma Metropolitana-Iztapalapa, Mexico City, Mexico
- Philip A. Gurnev, PhD, University of Massachusetts, Amherst, MA
- Jennifer C. Lee, PhD, Biochemistry and Biophysics Center, NHLBI, Bethesda
- Ekaterina M. Nestorovich, PhD, The Catholic University of America, Washington, DC
- Sergei Y. Noskov, PhD, University of Calgary, Calgary, Canada
- Adrian Parsegian, PhD, University of Massachusetts Amherst, Amherst, MA
- Olga Protchenko, PhD, Liver Diseases Branch, NIDDK, Bethesda, MD
- Dan Sackett, PhD, Program in Physical Biology, NICHD, Bethesda, MD
- Gerhard Wagner, PhD, Harvard Medical School, Cambridge, MA
- Michael Weinrich, MD, National Center for Medical Rehabilitation Research, NICHD, Bethesda, MD
- David L. Worcester, PhD, National Institute of Standards and Technology, Gaithersburg, MD
- Joshua Zimmerberg, MD, PhD, Program in Physical Biology, NICHD, Bethesda, MD
Contact
For more information, email bezrukov@helix.nih.gov or visit http://smt.nichd.nih.gov.