Membrane Rearrangements in Cell Entry by Cationic Peptides and in Cell-Cell Fusion
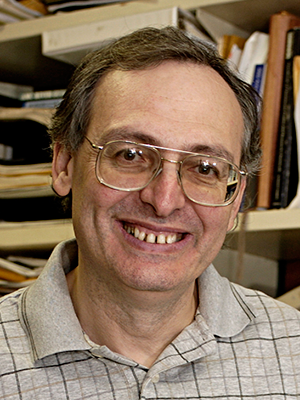
- Leonid V. Chernomordik, PhD, Head, Section on Membrane Biology
- Eugenia Leikina, DVM, Senior Research Assistant
- Kamram Melikov, PhD, Staff Scientist
- Elena Zaitseva, PhD, Research Fellow
- Santosh K. Verma, PhD, Visiting Fellow
- Berna Uygur, PhD, Postdoctoral Intramural Research Training Award Fellow
Diverse membrane remodeling reactions are tightly controlled by protein machinery but are also dependent on the lipid composition of membranes. Whereas each kind of protein has its own individual characteristics, membrane lipid bilayers have rather general properties manifested by their resistance to disruption and bending. Our long-term goal is to understand how proteins break and reseal membrane lipid bilayers in important cell biology processes, such as membrane fusion and crossing of cell membranes by water-soluble drugs on their way to intracellular targets. We expect that the analysis of the molecular mechanisms of different membrane rearrangements will clarify the generality of emerging mechanistic insights. Better understanding of these mechanisms will bring about new ways of controlling them and lead to new strategies for treating diseases involving cell invasion by enveloped viruses, intracellular trafficking, and intercellular fusion. In addition to our on-going work on myoblast fusion and osteoclast fusion, in recent studies we focused on the mechanisms underlying cell entry by cell-penetrating cationic peptides. We identified a highly efficient intracellular calcium–regulated pathway of cationic peptide entry into the cytosol and nucleus of adherent cells. Specific mechanisms by which cationic peptides activate intracellular signaling pathways and then cross the plasma membrane remain to be clarified. A better understanding of these mechanisms may enhance our knowledge of the properties of plasma membrane as well as guide development of future drug delivery vehicles.
Efficient entry of the cell-penetrating peptide nona-arginine into adherent cells involves a transient increase in intracellular calcium.
Macromolecular drugs such as polynucleotides, their mimics (peptide nucleic acids and phosphorodiamidate morpholino oligomers), bioactive peptides, and full-length proteins have great potential in the treatment of various diseases. Rapid development of such drugs is greatly facilitated by progress in biotechnology and by better understanding of the molecular mechanisms of pathogenesis. However, delivery of macromolecular drugs to their intracellular targets, which often reside in the cell cytosol or nucleus, remains challenging, because, along the way, several permeability barriers must be overcome, including a cell membrane that is generally impermeable to most macromolecules. Cationic cell-permeable peptides (CCPPs) are a promising delivery vehicle for a wide range of potential macromolecular drugs and have been successfully used to deliver various macromolecular cargo molecules both in vitro and in vivo. Over the past two decades, significant progress has been made in identifying and designing new CCPPs. In contrast, a clear understanding of the mechanisms by which CCPPs reach their targets in the cytosol and nucleus is still lacking. Most importantly, we still do not know how highly charged CCPPs and their cargo cross cell membranes (plasma membrane or endosomal membrane), which are presumed to present a non-permeable barrier, enter the cytosol and nucleus. This greatly hampers rational design of new CCPPs.
In earlier studies, we and others showed that, at 37°C, various pathways of endocytosis are involved in CCPPs' entry into cells. It has been suggested that endosome acidification and/or changes in lipid composition of the endosome upon maturation play an important role in CCPP escape. Regardless of the mechanism, the efficiency of endosomal escape is very low, with most of the peptide (and associated cargo) trapped in endosomes and then degraded. In search for more efficient ways of drug delivery into the cytosol and nucleus, we unexpectedly found that the rapid transfer of cells into cold medium (15°C) in the presence of the prototypical CCPP R9 (1–2μM) dramatically boosts the efficiency of R9 entry into the cytosol and nucleus, detected as nuclear labeling by rhodamine-tagged R9 (Figure 1). The amount of the peptide entering an individual cell at 15°C within 15 min was at least 50 times higher than the amount of the peptide entering a cell within 40 min at 37°C by endocytosis. Similarly, efficient entry of CCPPs into cytosol and nucleus can be induced by high concentrations of the peptide (10μM or more at 37°C). Importantly, such efficient entry is limited to the cationic peptide and is not accompanied by the entry of concomitantly added small membrane-impermeable dyes such as calcein or SYTOX Green. Intriguingly, whether induced by temperature drop or by a high CCPP concentration, we could detect peptide entry into cytosol and nucleus only in a sub-population of cells, suggesting an active role of the cell in the entry process. Indeed, both entry pathways were inhibited by depletion of intracellular ATP. We also observed an intriguing dependence of both pathways on a rise in intracellular Ca2+ concentration. In particular, addition of high concentrations of the peptide to the cells induced repetitive transient increases in intracellular Ca2+ concentration. Interestingly, we observed a significant variation in cell response to the peptide, with repetitive spikes of intracellular Ca2+ noted in some but not all cells within the same observation field. Importantly, there was a significant correlation between CCPP entry and appearance of Ca2+ spikes. Inhibition of both pathways of peptide entry by a chelator of intracellular Ca2+ further confirmed the role of the intracellular Ca2+ and suggested involvement of intracellular signaling pathways in the process. Using chemical inhibitors, we also demonstrated that both entry of Ca2+ from extracellular medium and release of Ca2+ from intracellular stores play an important role in the mechanism of CCPP entry.
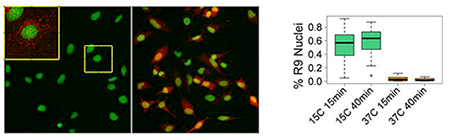
Click image to enlarge.
Temperature drop induces efficient entry of Rhodamine-labeled R9 peptide (Rh-R9) into HeLa cells.
A. Cells were incubated with 2mM of Rh-R9 (red) for 15 min at 37°C or 15°C, washed, and imaged immediately without fixation. Nuclei are labeled with Hoechst (green). Inset shows an enlargement of the highlighted area with Rh signal amplified to demonstrate endocytic entry. B. Fraction of cells with Rh-labeled nuclei after 15 min or 40 min incubation at 15°C or 37°C. Boxplots show the median as central line, 1st and 3rd quartiles as edges of the box, range as whiskers, and outliers as symbols.
Taken together, our data indicate that interactions of CCPPs with cells activate intracellular signaling cascades that result in significant changes in plasma membrane permeability for highly cationic peptides. Importantly, we demonstrated that cells do not act as mere targets for drug delivery but may actively interact with the added drug conjugate. Clearly, the side effects of the drug on cell metabolism should be taken into account in designing future CCPPs but may also be useful. For example, manipulation of intracellular calcium levels with drugs could be used to promote entry of the CCPPs. Indeed, we demonstrated that flulfenamic acid (a member of the anthranilic acid derivatives class of nonsteroidal anti-inflammatory drugs) induces entry of R9 at 37°C at a peptide concentration (2μM) that does not induce an increase in the intracellular calcium level and at which no entry of the peptide into cytosol/nucleus is observed when peptide is added alone. These 'proof of principle' experiments underscore the importance of our discovery for development of new drug delivery vehicles and protocols.
Modulation of plasma membrane permeability for highly cationic peptides by intracellular calcium signaling is an important new phenomenon that deserves further investigation. Of the many important questions that await an answer, we highlight just a few, such as how interactions of the CCPP with plasma membrane induce increase in intracellular Ca2+ concentration. It is possible that cell surface–bound cationic peptides electrostatically modulate activity of proximal plasma-membrane cation channels. Indeed, we previously reported that binding of R9 to negatively charged lipids strongly modulates conductance of the gramicidin A channel reconstituted into black lipid membranes. Alternatively, direct interaction of the peptide with cell-surface receptors may lead to their activation and to the opening of associated Ca2+ channels. A better understanding of this first step should provide guidance for the design of more efficient CCPPs but can also provide valuable insight into the cellular interactions of other important cationic peptide, defensins being one example. The specifics of calcium signaling pathways that lead to an increase in plasma membrane permeability to CCPPs is another largely unexplored but very important area. In our work, using phosphatidylserine-binding domain of lactadherin, we demonstrated that Ca2+-dependent cell-surface exposure of phosphatidylserine, a lipid normally residing only in the inner leaflet of the plasma membrane, is involved both in temperature- and high concentration–induced entry of R9. However, the precise mechanistic role of the phosphatidylserine cell-surface exposure in R9 entry remains to be clarified. We expect that better delineation of the specific activation sequences involved in Ca2+-dependent peptide entry will suggest new, more specific, and efficient approaches to induce CCPP entry. The mechanism of plasma membrane permeability to cationic peptide remains elusive, in particular, whether it is mediated through changes in lipid composition (perhaps surface exposure of phosphatidylserine is one of them) or involves some protein channels or transporters. Addressing this question is important not only for the drug delivery field but more broadly for understanding the transport properties of the plasma membrane.
Additional Funding
- United States - Israel Binational Science Foundation (BSF) grant “Machinery of myoblast fusion” 2015-2019
Publications
- Uygur B, Abramo K, Leikina E, Vary C, Liaw L, Wu WS. SLUG is a direct transcriptional repressor of PTEN tumor suppressor. Prostate 2015; 75:907-916.
- Kozlov MM, Chernomordik LV. Membrane tension and membrane fusion. Curr Opin Struct Biol 2015; 33:61-67.
- Melikov K, Hara A, Yamoah K, Zaitseva E, Zaitsev E, Chernomordik LV. Efficient entry of cell-penetrating peptide nona-arginine into adherent cells involves a transient increase in intracellular calcium. Biochem J 2015; 471:221-230.
- Chernomordik LV, Kozlov MM. Myoblast fusion: playing hard to get. Dev Cell 2015; 32:529-530.
- Zicari S, Arakelyan A, Fitzgerald W, Zaitseva E, Chernomordik LV, Margolis L, Grivel J-Ch. Evaluation of the maturation of individual dengue virions with flow virometry. Virology 2015; 488:20-7.
Collaborators
- Anush Arakelyan, PhD, Program in Physical Biology, NICHD, Bethesda, MD
- Aurelia Defour, PhD, Children’s National Medical Center, Washington, DC
- Claudia M. Gebert, PhD, Program in Genomics of Differentiation, NICHD, Bethesda, MD
- Jyoti Jaiswal, PhD, George Washington University School of Medicine and Health Sciences, Washington, DC
- Michael M. Kozlov, PhD, DHabil, Sackler Faculty of Medicine, Tel Aviv University, Tel Aviv, Israel
- Leonid Margolis, PhD, Program in Physical Biology, NICHD, Bethesda, MD
- Karl Pfeifer, PhD, Program in Genomics of Differentiation, NICHD, Bethesda, MD
- Benjamin Podbilewicz, PhD, Technion-Israel Institute of Technology, Haifa, Israel
- Calvin P. Vary, PhD, Maine Medical Center Research Institute, Scarborough ME
- Wen-Shu Wu, PhD, University of Illinois College of Medicine at Chicago, Chicago, IL
- Sonia Zicari, PhD, Program in Physical Biology, NICHD, Bethesda, MD
Contact
For more information, email chernoml@mail.nih.gov or visit irp.nih.gov/pi/leonid-chernomordik.