Extracellular Matrix Disorders: Molecular Mechanisms and Treatment Targets
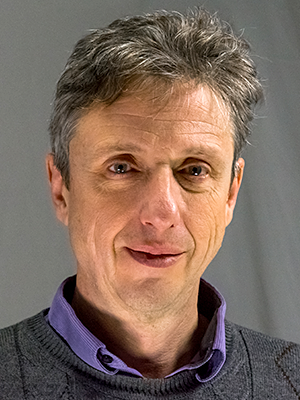
- Sergey Leikin, PhD, Head, Section on Physical Biochemistry
- Elena N. Makareeva, PhD, Staff Scientist
- Edward L. Mertz, PhD, Staff Scientist
- Shakib Omari, PhD, Postdoctoral Fellow
- Katrina Y. Koon, BS, Postbaccalaureate Fellow
- Lynn S. Mirigian (Felts), BS, Predoctoral Fellow (Graduate Student)
The extracellular matrix (ECM) is involved in a wide variety of disorders, ranging from rare genetic abnormalities of skeletal development (skeletal dysplasias) to such common ailments as osteoporosis, fibrosis, and cancer. Our interest in ECM biology began with studies on basic principles relating the helical structure of collagen and DNA to their interactions and biological function. Over the years, the focus of our research shifted to collagens, which are the most abundant ECM molecules, and then to ECM disorders and the development of novel treatments for these disorders. We gradually phased out the DNA studies and concentrated on ECM pathology in cancer, fibrosis, osteogenesis imperfecta, Ehlers-Danlos syndrome, chondrodysplasias, osteoporosis, and other diseases. Together with other NICHD and extramural clinical scientists, we strive to improve our knowledge of the molecular mechanisms underlying those diseases. We hope to use the knowledge gained through our studies for diagnostics, characterization, and treatment, bringing our expertise in physical biochemistry and theory to clinical research and practice.
Procollagen folding and its role in bone disorders
Collagens are triple-helical proteins forming structural scaffolds that hold together bone, cartilage, skin, and other tissues. In bone, they are produced by osteoblasts, the cells responsible for synthesis and mineralization of new bone material. By far the most abundant proteins in all vertebrates, collagens account for up to 30% of all proteins synthesized by osteoblasts. Yet, folding of their procollagen precursors within the endoplasmic reticulum (ER) presents an extraordinary challenge for cells. We discovered that the equilibrium state of type I procollagen in the absence of chaperones is a random coil. Folding of the human procollagen triple helix in an ER–like environment is favorable below 35°C but not at normal body temperature. At this temperature, natively folded collagen molecules become stable only after incorporation into fibers. When not incorporated, individual molecules denature within several hours and become susceptible to rapid proteolytic degradation. To overcome such intrinsic instability, cells must use specialized chaperones to fold the triple helix. It is not completely clear which chaperones are involved in this process, how they function, and how cells recognize properly folded procollagen and handle it when misfolded. Because of massive procollagen synthesis, osteoblast malfunction caused by procollagen misfolding might be an important factor in bone pathologies. For instance, osteogenesis imperfecta (OI) appears to be primarily a procollagen misfolding disease (References 1 and 2). Moreover, an inability of aging osteoblasts to handle normal procollagen folding load might also contribute to common osteoporosis. Understanding procollagen folding and the cellular response to its misfolding is therefore important both from fundamental and practical perspectives.
The most common mutations affecting procollagen folding are Gly substitutions in the obligatory (Gly-X-Y)n sequence of the triple helix. Such substitutions in type I collagen are responsible for approximately 80% of severe OI cases. The substitutions' effects on procollagen folding are determined by the location and identity of the substituting residue. Our studies of collagens from OI patients with over 50 different Gly substitutions revealed several structural regions within the triple helix where mutations might be responsible for distinct OI phenotypes. For example, the first 85–90 amino acids at the N-terminal end of the triple helix form an “N-anchor” domain with higher than average triple helix stability. Gly substitutions within this region disrupt the whole N-anchor, preventing normal cleavage of the adjacent N-propeptide. Incorporation of collagen with uncleaved N-propeptides into fibrils leads to hyperextensibility and joint laxity more characteristic of the Ehlers-Danlos syndrome (EDS). α1(I) Gly substitutions within another region, which surrounds the collagenase cleavage site, might be lethal because the sequence of this region prevents efficient renucleation of normal C-to-N-terminus helix folding, once the mutation is encountered.
At present, we are focusing on understanding how cells respond to misfolding of procollagen with different Gly substitutions. We hypothesize that unconventional folding requirements produce an unconventional cell stress response to accumulation of misfolded procollagen. In collaboration with Peter Byers, we found that Gly substitutions cause slower folding as well as misfolding of procollagen, its accumulation in the ER of cultured patient fibroblasts, ER dilation, and activation of a cell stress response reminiscent of the "ER Overload" associated with misfolding of serpin-family proteins in serpinopathies. The observations provided an important clue to OI pathophysiology and highlighted autophagy as a potentially important therapeutic target.
To further pursue molecular mechanisms of the cell stress response and its targeting, we are investigating a mouse OI model with a Gly610 to Cys substitution in the triple helical region of the α2(I) chain. The G610C mouse mimics the mutation found in a large group of related patients. Our study of cultured fibroblasts and osteoblasts as well as tissues in this model revealed misfolding and accumulation of mutant molecules in the ER similar to human patient fibroblasts. Increased phosphorylation of EIF2α indicated the presence of cell stress response, yet we found no evidence of conventional unfolded protein response (UPR) signaling. We found that misfolded procollagen molecules are degraded by lysosomes via autophagy, rather than by proteasomes via ER–associated degradation. Osteoblasts adapt to the misfolding of mutant molecules and eliminate them by enhancing the autophagy. However, the adaptation occurs at the cost of abnormal cell function, e.g., blunted response to stimulation of collagen production by TGF-β and abnormal Wnt signaling. Abnormal function of the latter pathways likely contributes to the abnormal osteoblast differentiation and maturation observed in G610C animals. For instance, the animals exhibit delayed bone mineralization during early development, and their cultured bone marrow stromal cells (BMSCs) form fewer colonies capable of producing mineralized matrix. As an adaptation to deficient osteoblast maturation, G610C mice generate more osteoblastic cells. However, each mutant cell produces much less bone than its normal counterpart. The combination of increased bone formation surfaces with reduced bone formation rate at these surfaces disrupts normal bone modeling, causing, e.g., long-term entrapment of poorly organized woven bone between layers of lamellar bone. The resulting disruption of the cortical bone matrix structure leads to more brittle bones, which have a heightened susceptibility to fracture upon high energy impact despite normal cortical thickness and slightly increased cortical bone mineral density.
Development of novel OI treatments
We hypothesize that, by enhancing autophagy, it might be possible to prevent excessive accumulation of misfolded procollagen molecules in the cell, reducing cell stress and thereby improving osteoblast differentiation and function. As an initial approach to testing the hypothesis, we placed G610C mice on a low protein diet from 8 to 17 weeks of age, which partially normalized mineralization of newly deposited cortical bone and improved BMSC differentiation in culture. However, the positive effects were counterbalanced by reduced animal growth and less deposition of new bone. Overall, our preliminary data suggest that autophagy enhancement might be a useful therapeutic strategy, but a continuous low protein diet is not likely to be a suitable approach, particularly in pediatric OI patients.
Surprisingly, BMSCs appeared to "remember" the animal's diet for up to several weeks at identical cell culture conditions. Apparently, the diet produced some epigenetic changes, e.g., resulting in enhanced autophagy even after cell removal from animals and prolonged culture, suggesting that an intermittent low protein diet might be useful for alleviating osteoblast cell stress without reducing bone deposition. However, diet affects multiple physiological functions. While the dietary treatment is easy to implement, the mechanisms underlying its effects are difficult to interpret, and even an intermittent low-protein diet may have a variety of unintended long-term consequences. It is therefore important to understand how bone formation and underlying osteoblast differentiation and function are affected by more specifically targeted autophagy manipulation that has no, or at least fewer, off-target effects.
To address this question, we generated G610C mice, in which autophagy can be repressed or activated by conditional targeted knock-out or overexpression of the Atg5 gene in cells of osteoblast lineage. In an ongoing study of these animals, we found that knock-out of both Atg5 alleles in mature osteoblasts significantly worsens the bone phenotype. This initial observation supports the importance of autophagy in the animal adaptation to mutant procollagen misfolding, but validation of autophagy as a useful therapeutic target requires testing the effects of its osteoblast-specific enhancement. We are currently completing animal breeding for these experiments, which we hope will provide a better understanding of the role of autophagy in normal function and pathology of osteoblasts as well as identify novel targets and therapeutic approaches to OI and other collagen-misfolding disorders.
Translational studies of patients with novel or unusual OI and EDS mutations
Abnormal collagen biosynthesis and malfunction of osteoblasts are also important factors in OI caused by other collagen mutations as well as by mutations in other proteins. Over the last several years, we assisted several clinical research groups in characterizing collagen biosynthesis and folding in fibroblasts from patients with newly discovered recessive forms of OI and closely related skeletal dysplasia caused by mutations in cartilage-associated protein (CRTAP), prolyl-3-hydrohylase (P3H1), cyclophilin B (CYPB), FKBP65, WNT1, and TRICB. In particular, our collaboration with Joan Marini suggested that the CRTAP/P3H1/CYPB complex functions as a procollagen chaperone. A deficiency in any of the three proteins delays procollagen folding, although their exact role in procollagen folding remains unclear (Reference 3). More surprisingly, we found no detectable changes in the procollagen folding rate in cultured fibroblasts from patients with FKBP65 mutations. Our data suggest that FKBP65 may affect post-translational modification of procollagen and deposition of collagen matrix by a different mechanism. It remains unclear why some FKBP65 mutations cause severe OI with joint contractures (Bruck syndrome) while others cause joint contractures without pronounced OI (Kuskokwim syndrome) or OI without pronounced joint contractures.
More recently, in collaboration with Joan Marini, we investigated collagen and ECM biosynthesis abnormalities caused by deficiencies in the transmembrane protein TRICB in fibroblasts from Bedouin OI patients in Israel and Saudi Arabia. The study revealed unusual underhydroxylation of lysine residues in collagen chains, in contrast to overhydroxylation of lysine caused by most other OI mutations, as well as secretion of 30–40% of procollagen molecules with abnormal conformation and reduced thermal stability. Given that TRICB is involved in Ca2+ release from the ER and that its absence causes abnormal Ca2+ concentrations and flux in patient cells, OI in these patients may be caused by dysregulation of several collagen-specific chaperones and modifying enzymes in the ER through Ca2+ modulation. However, TRICB may also have a more direct chaperone function in procollagen folding. We also assisted Paul Coucke’s laboratory in characterization of procollagen biosynthesis abnormalities in a complex lethal osteochondrodysplasia caused by defective TAPT1 protein (Reference 4).
Most single amino acid substitutions in X and Y positions of the repeating Gly-X-Y collagen sequence are considered to be neutral variants. Several Arg-to-Cys substitutions were recently reported, but it remained unclear whether the resulting OI and EDS were caused by aberrant disulfide bonds formed in the ECM by the Cys residue, which is not present in normal type I collagen, or by abnormal biosynthesis of mutant molecules. In collaboration with Peter Byers, we analyzed collagen-metabolism pathology caused by substitutions of the same Y-position Arg780 in the α1(I) chain to Leu and Cys. The study revealed that the loss of Y-position Arg causes abnormal procollagen folding by reducing triple helix stability and eliminating important HSP47–binding sites, resulting in accumulation of misfolded procollagen in the ER and ER dilation similar to the effects of Gly substitutions. These effects are likely responsible for the bone fragility caused by Y- but not X-position Arg substitutions, while aberrant Cys disulfide bonds likely contribute to EDS in patients with either Y- or X-position substitutions.
Extracellular matrix pathology in tumors and fibrosis
Another important advance from our work of the past several years was the characterization of a collagenase-resistant, homotrimeric isoform of type I collagen and its potential role in cancer, fibrosis, and other disorders. The normal isoform of type I collagen is a heterotrimer of two α1(I) chains and one α2(I) chain. Homotrimers of three α1(I) chains are produced in some fetal tissues, carcinomas, fibrotic tissues, as well as in rare forms of OI and EDS associated with α2(I) chain deficiency. We found the homotrimers to be at least 5–10 times more resistant to cleavage by all mammalian collagenases than the heterotrimers, and we determined the molecular mechanism of this resistance. Our studies suggested that cancer cells might utilize the collagen isoform to build collagenase-resistant tracks, thus supporting invasion through stroma of lower resistance.
We also collaborated with Constantine Stratakis's lab to investigate bone tumors caused by defects in protein kinase A (PKA), a key enzyme in the cAMP signaling pathway. Initially, we investigated synthesis of type I collagen homotrimers. However, over the last three years, the focus of the study has shifted to abnormal differentiation of osteoblastic cells and deposition of bone within these tumors (Reference 5). We found that knockouts of various PKA subunits cause not only abnormal organization and mineralization of bone matrix but also novel bone structures that had not been previously reported. For instance, we observed free-standing cylindrical bone spicules with osteon-like organization of lamellae and osteocytes but an inverted mineralization pattern, a highly mineralized central core, and decreasing mineralization away from the central core. Currently, we are assisting the Stratakis lab in characterizing abnormal osteoblast maturation, the role of an abnormal inflammatory response, and effects of anti-inflammatory drug treatments in these animals. Improved understanding of bone tumors caused by PKA deficiencies may not only clarify the role of cAMP signaling but also suggest new approaches to therapeutic manipulation of bone formation in skeletal dysplasias.
Multi-modal micro-spectroscopic imaging and mapping of tissues
Label-free micro-spectroscopic infrared and Raman imaging of tissues and cell cultures provides important information about the chemical composition, organization, and biological reactions inaccessible by traditional histology. However, applications of these techniques were severely restricted by light-path instabilities in thin hydrated specimens under physiological conditions. We resolved the problem by designing specimen chambers with precise thermo-mechanical stabilization for high-definition (HD) infrared imaging and Raman micro-spectroscopy, achieving spectral reproducibility up to two orders of magnitude better than in leading commercial instruments. The HD technology was essential for analysis of abnormal collagen matrix deposition by CRTAP– and FKBP65–deficient cells. It has enabled us to assist NIBIB scientists in characterizing a functionalized carbon-nanotube approach to the delivery of anticancer agents into cells that overexpress hyaluronate receptors and is crucial for our current studies of bone structure and mineralization in the mouse models of OI and PKA deficiencies described above.
The power of the technology is best illustrated by our studies of ECM structure and composition effects on the function of cartilage in a mouse model of diastrophic dysplasia (DTD) caused by mutations in the SLC26A2 sulfate transporter, deficient sulfate uptake by chondrocytes, and resulting under-sulfation of glycosaminoglycans in cartilage matrix. In collaboration with Antonella Forlino and Antonio Rossi, we found that the deficiency results in under-sulfation of chondroitin and disorientation of collagen fibers, disrupting a thin protective layer at the articular surface and causing subsequent cartilage degradation. We investigated the relationship between chondroitin under-sulfation and the rate of its synthesis across the growing epiphyseal cartilage and built a mathematical model for the sulfation pathway, predicting treatment targets for sulfation-related chondrodysplasias and genes that might contribute to the juvenile idiopathic arthritis recently associated with single nucleotide polymorphisms in the SLC26A2 transporter.
We are extending the technology by combining imaging of bone and cartilage ECM composition and structure with biomechanical measurements at the same length scales. The mechanical properties of bone and cartilage should depend on the deformation length scale because of the heterogeneous microscopic structure and the presence of different macroscopic regions and zones in these tissues. Nevertheless, biomechanical studies are rarely accompanied by mapping of tissue composition and structure. To address the problem, we are collaborating with Peter Basser and Emilios Dimitriadis on mapping cartilage elasticity by force microscopy at length scales appropriate for examining the material properties of the ECM and on combining it with our multimodal imaging technology.
While the ECM plays a key role in normal development and pathology of all tissues, most studies focus on expression of its components rather than its overall organization. Our multimodal imaging technology is helping to close this gap in in vitro studies of tissue sections and cell cultures. To translate these advances into clinical practice, we established a new collaboration with Peter Basser on utilizing the technology to calibrate and test newer methods for noninvasive in vivo ECM studies by the solid state magnetic resonance imaging (MRI) that is being developed in his laboratory.
Currently, in collaboration with Peter Basser and Roberto Romero, we are adapting HD infrared and Raman technologies for quantitative imaging of structural organization, composition and mechanical properties of ECM in the normal and pathological human placenta. Our initial measurements have already identified several previously unreported features of placental ECM: (1) highly anisotropic organization of fibrin and collagen, which may depend on and affect the blood flow and determine the tissue strength; (2) deposition of cholesteryl ester aggregates in fibrin fibrinoids from maternal blood; and (3) formation of collagen “fibroids” and high collagen content in matrix fibrinoids. In addition to identifying novel features of placental ECM organization, we are utilizing the HD infrared and Raman imaging to guide detection of ECM abnormalities in the same sample by single-sided (SS-NMR) and magnetization exchange (MEX-MRI) NMR/MRI methods, for subsequent application in noninvasive real-time imaging of human placenta pathology.
Additional Funding
- NICHD Director's Award (2014): Targeting Autophagy of Misfolded Molecules in Collagen-Related Dysplasias
- NICHD Director's Human Placenta Award (2015): Imaging Extracellular Matrix in Human Placenta
Publications
- Makareeva E, Leikin S. Collagen structure, folding and function. Osteogenesis Imperfecta: A translational approach to brittle bone disease. Eds: Shapiro et al., Academic Press 2014; 71-84.
- Mirigian LS, Makareeva E, Leikin S. Pulse-chase analysis of procollagen biosynthesis by azidohomoalanine labeling. Connect Tissue Res 2014; 55(5-6):403-410.
- Cabral WA, Perdivara I, Weis M, Terajima M, Blissett AR, Chang W, Perosky JE, Makareeva EN, Mertz EL, Leikin S, Tomer KB, Kozloff KM, Eyre DR, Yamauchi M, Marini JC. Abnormal type I collagen post-translational modification and crosslinking in a cyclophilin B KO mouse model of recessive osteogenesis imperfecta. PLoS Genetics 2014; 10:e1004465.
- Symoens S, Barnes AM, Gistelinck C, Malfait F, Guillemyn B, Steyaert W, Syx D, D'Hondt S, Biervliet M, De Backer J, Witten EP, Leikin S, Makareeva E, Gillessen-Kaesbach G, Huysseune A, Vleminckx K, Willaert A, De Paepe A, Marini JC, Coucke PJ. Genetic defects in TAPT1 disrupt ciliogenesis and cause a complex lethal osteochondrodysplasia. Am J Hum Genet 2015; 97:521-534.
- Liu S, Saloustros E, Mertz EL, Tsang K, Starost MF, Salpea P, Faucz FR, Szarek E, Nesterova M, Leikin S, Stratakis CA. Haploinsufficiency for either one of the type-II regulatory subunits of protein kinase A improves the bone phenotype of Prkar1a+/- mice. Hum Mol Genet 2015; 24:6080-6092.
Collaborators
- Peter Basser, PhD, Program on Pediatric Imaging and Tissue Sciences, NICHD, Bethesda, MD
- Peter H. Byers, MD, University of Washington, Seattle, WA
- Paul J. Coucke, PhD, Ghent University Hospital, Ghent, Belgium
- Sarah Dallas, PhD, University of Missouri, Kansas City, MO
- Emilios K. Dimitriadis, PhD, Biomedical Engineering & Physical Science Shared Resource Program, NIBIB, Bethesda, MD
- Motomi Enomoto-Iwamoto, DDS, PhD, University of Pennsylvania, Philadelphia, PA
- Antonella Forlino, PhD, Università degli Studi di Pavia, Pavia, Italy
- Kenn Holmbeck, PhD, Matrix Metalloproteinase Section, NIDCR, Bethesda, MD
- Ken Kozloff, PhD, University of Michigan, Ann Arbor, MI
- Jennifer A. Lippincott-Schwartz, PhD, Cell Biology and Metabolism Program, NICHD, Bethesda, MD
- Joan C. Marini, MD, PhD, Bone and Extracellular Matrix Branch, NICHD, Bethesda, MD
- George Patterson, PhD, Section on Biophotonics, NIBIB, Bethesda, MD
- Charlotte L. Phillips, PhD, University of Missouri, Columbia, MO
- Pamela G. Robey, PhD, Craniofacial and Skeletal Diseases Branch, NIDCR, Bethesda, MD
- Roberto Romero, MD, Program in Perinatal Research and Obstetrics, NICHD, Detroit, MI
- Antonio Rossi, PhD, Università degli Studi di Pavia, Pavia, Italy
- Dan L. Sackett, PhD, Program in Physical Biology, NICHD, Bethesda, MD
- Constantine A. Stratakis, MD, DMedSci, Program on Developmental Endocrinology and Genetics, NICHD, Bethesda, MD
Contact
For more information, email leikins@mail.nih.gov or visit http://physbiochem.nichd.nih.gov.