Studies on DNA Replication, Repair, and Mutagenesis in Eukaryotic and Prokaryotic Cells
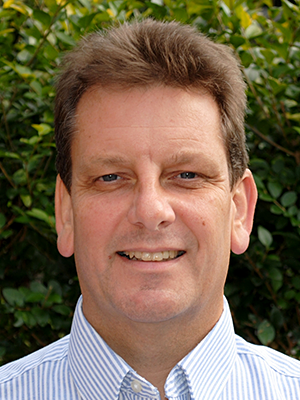
- Roger Woodgate, PhD, Chief, Laboratory of Genomic Integrity
- Ekaterina Chumakov, PhD, Staff Scientist
- Alexandra Vaisman, PhD, Interdisciplinary Scientist
- John McDonald, PhD, Biologist
- Mary McLenigan, BS, Chemist
- Ewa Grabowska, PhD, Postdoctoral Visiting Fellow
- Ashley Swanson, PhD, Postdoctoral Intramural Research Training Award Fellow
- Erin Walsh, PhD, Postdoctoral Intramural Research Training Award Fellow
- David Wilson, BS, Postbaccalaureate Intramural Research Training Award Fellow
- Donald Huston, BS, Technical Intramural Research Training Award Trainee
- Hallie Whalen, Student Fellow
Under optimal conditions, the fidelity of DNA replication is extremely high. Indeed, it is estimated that, on average, only one error occurs for every 10 billion bases replicated. However, given that living organisms are continually subjected to a variety of endogenous and exogenous DNA–damaging agents, optimal conditions rarely prevail in vivo. While all organisms have evolved elaborate repair pathways to deal with such damage, the pathways rarely operate with 100% efficiency. Thus, persisting DNA lesions are replicated, but with much lower fidelity than in undamaged DNA. Our aim is to understand the molecular mechanisms by which mutations are introduced into damaged DNA. The process, commonly referred to as translesion DNA synthesis (TLS), is facilitated by one or more members of the Y-family of DNA polymerases, which are conserved from bacteria to humans. Based on phylogenetic relationships, Y-family polymerases may be broadly classified into five subfamilies: DinB-like (polIV/pol kappa–like) proteins are ubiquitous and found in all domains of life; in contrast, the Rev1-like, Rad30A (pol eta)–like, and Rad30B (pol iota)–like polymerases are found only in eukaryotes, and the UmuC (polV)–like polymerases only in prokaryotes. We continue to investigate TLS in all three domains of life: bacteria, archaea, and eukaryotes.
Prokaryotic DNA repair and mutagenesis
Most damage-induced mutagenesis in Escherichia coli is dependent upon the UmuD′C protein complex, which comprises DNA polymerase V (pol V). Pol V has intrinsically weak catalytic activity, but is dramatically stimulated by interactions with ATP and the bacterial recombinase RecA to form a functional complex called the DNA/pol V mutasome (pol V Mut). In a collaborative study with Michael Cox and Myron Goodman, we recently identified the protein-protein interface between UmuC and RecA that is critical for pol V activation (Reference 1). This was achieved by using mutant RecA proteins, a range of in vitro and in vivo approaches, and a photo-activated unnatural amino acid at RecA N113.
In particular, we identified a RecA surface defined by residues 112–117 that either directly interacts with, or is in very close proximity to, amino acid residues on two distinct surfaces of the UmuC subunit of pol V. One surface is uniquely prominent in the active pol V Mut. Several conformational states are populated in the inactive and active complexes of RecA with pol V. The RecA D112R and RecA D112R N113R double mutant proteins exhibit successively reduced capacity for pol V activation. The double mutant RecA is specifically defective in the ATP–binding step of the activation pathway. Unlike the classic non-mutable RecA S117F (recA1730), the RecA D112R N113R variant exhibits no defect in filament formation on DNA and promotes all other RecA activities efficiently. An important pol V activation surface of RecA protein is thus centered in a region encompassing amino acid residues 112, 113, and 117, a surface exposed at the 3′-proximal end of a RecA filament. The same RecA surface is not utilized in the RecA activation of the homologous and highly mutagenic RumA′2B polymerase encoded by the integrating-conjugative element (ICE) R391, indicating a lack of structural conservation between the two systems. The RecA D112R N113R protein therefore represents a new separation of function mutant, proficient in all RecA functions except SOS mutagenesis.
We extended our studies on the regulation of pol V through a collaboration with Antoine van Oijen and Andrew Robinson. In particular, we focused on the hitherto under-appreciated spatial regulation imposed on pol V in Escherichia coli (Reference 2). Spatial regulation is often encountered as a component of multi-tiered regulatory systems in eukaryotes, where processes are readily segregated by organelle boundaries. Well characterized examples of spatial regulation are much less common in bacteria. Given its mutagenic potential, pol V activity has previously been shown to be controlled by means of an elaborate regulatory system at both the transcriptional and post-translational levels. However, by using single-molecule fluorescence microscopy to visualize UmuC inside living cells in space and time, we demonstrated that pol V is also subject to a novel form of spatial regulation. After an initial delay (about 45 min) post UV irradiation, UmuC is synthesized, but is not immediately activated. Instead, it is sequestered at the inner cell membrane. The release of UmuC into the cytosol requires cleavage of UmuD to UmuD′ mediated by the nucleoprotein filament RecA* (active RecA filaments on single-stranded DNA). Classic SOS damage response mutants either block [umuD(K97A)] or constitutively stimulate [recA(E38K)] UmuC release from the membrane. Furthermore, foci of mutagenically active pol V Mut (UmuD′2C-RecA-ATP) formed in the cytosol after UV irradiation do not co-localize with pol III replisomes, suggesting the capacity to promote translesion DNA synthesis at lesions skipped over by DNA polymerase III. In effect, at least three molecular mechanisms limit the amount of time that pol V has to access DNA: (1) transcriptional and post-translational regulation that initially keep the intracellular levels of pol V to a minimum; (2) spatial regulation via transient sequestration of UmuC at the membrane, which further delays pol V activation; and (3) the hydrolytic activity of a recently discovered pol V Mut ATPase function that limits active polymerase time on the chromosomal template.
Eukaryotic DNA repair and mutagenesis
Our studies on the eukaryotic TLS polymerases focused on Saccharomyces cerevisiae polymerase eta (pol eta) and human polymerase iota (pol iota). Pol eta is best characterized for its ability to perform accurate and efficient TLS through cyclobutane pyrimidine dimers (CPDs). To ensure accurate bypass, the polymerase is not only required to select the correct base but also discriminate between NTPs and dNTPs. Most DNA polymerases have a conserved 'steric gate' residue, which functions to prevent incorporation of NMPs during DNA synthesis. In our collaborative study with Susana Cerritelli and Robert Crouch, we demonstrated that the Phe35 residue of S. cerevisiae pol eta functions as a steric gate to limit the use of ribonucleotides during polymerization both in vitro and in vivo (Reference 3). Unlike the related pol iota enzyme, wild-type pol eta does not readily incorporate NMPs in vitro. In contrast, a pol eta F35A mutant incorporates NMPs on both damaged and undamaged DNA in vitro with a high degree of base selectivity. An S. cerevisiae strain expressing pol eta F35A (rad30-F35A) that is also deficient for nucleotide excision repair (rad1delta) and the TLS polymerase pol zeta (rev3delta) are extremely sensitive to UV light. The sensitivity is attributable, in part, to RNase H2 activity, as an isogenic rnh201delta strain is roughly 50-fold more UV–resistant than its RNH201+ counterpart. Interestingly the rad1delta rev3delta rad30-F35A rnh201delta strain exhibits a significant increase in the extent of spontaneous mutagenesis with a spectrum dominated by 1 bp deletions at runs of template thymine nucleotides. We hypothesized that the increased mutagenesis is the result of adenine ribonucleotide (rA) incorporation at these sites and that the short poly rA tract is subsequently repaired in an error-prone manner by a novel repair pathway that is specifically targeted to polyribonucleotide tracks. These data indicate that, under certain conditions, pol eta can compete with the cell’s replicases and gain access to undamaged genomic DNA. Such observations are consistent with a role for pol eta in replicating common fragile sites (CFS) in human cells.
Our studies on the human TLS polymerases focused on how they are regulated by post-translational modification. Indeed, both human pol eta and pol iota were previously shown to be monoubiquitinated in vivo (Reference 4). Pol eta was shown to be ubiquitinated at one primary site. When this site is unavailable, three nearby lysines may become ubiquitinated. In contrast, mass-spectrometry analysis of monoubiquitinated pol iota revealed that it is ubiquitinated at over 27 unique sites (Reference 5). Many of these sites are located in different functional domains of the protein, including the catalytic polymerase domain, the PCNA–interacting region, the Rev1–interacting region, as well as its Ubiquitin Binding Motifs, UBM1 and UBM2. Pol iota monoubiquitination remains unchanged after cells are exposed to DNA–damaging agents such as UV light (generating UV photoproducts), ethyl methanesulfonate (generating alkylation damage), mitomycin C (generating interstrand crosslinks), or potassium bromate (generating direct oxidative DNA damage). However, when exposed to naphthoquinones, such as menadione and plumbagin, which cause indirect oxidative damage through mitochondrial dysfunction, pol iota becomes transiently polyubiquitinated via K11– and K48–linked chains of ubiquitin and subsequently targeted for degradation. Polyubiquitination does not occur as a direct result of the perturbation of the redox cycle, as no polyubiquitination was observed after treatment with rotenone or antimycin A, which inhibit mitochondrial electron transport. Interestingly, polyubiquitination was observed after the inhibition of the lysine acetyltransferase KATB3/p300. We hypothesize that the formation of polyubiquitination chains attached to pol iota occurs via an interplay between lysine acetylation and ubiquitination of ubiquitin itself at K11 and K48 rather than oxidative damage per se.
Additional Funding
- NIH Director’s Challenge Award
- U01HD085531-01: Replisome dynamics in M. tuberculosis: linking persistence to genetic resistance
Publications
- Gruber AJ, Erdem AL, Sabat G, Karata K, Jaszczur MM, Vo DD, Olsen TM, Woodgate R, Goodman MF, Cox MM. A RecA protein surface required for activation of DNA polymerase V. PLoS Genet 2015; 11(3):e1005066.
- Robinson A, McDonald JP, Caldas VE, Patel M, Wood EA, Punter CM, Ghodke H, Cox MM, Woodgate R, Goodman MF, van Oijen AM. Regulation of mutagenic DNA polymerase V activation in space and time. PLoS Genet 2015; 11(8):e1005482.
- Donigan KA, Cerritelli SM, McDonald JP, Vaisman A, Crouch RJ, Woodgate R. Unlocking the steric gate of DNA polymerase eta leads to increased genomic instability in Saccharomyces cerevisiae. DNA Repair 2015; 35:1-12.
- McIntyre J, McLenigan MP, Frank EG, Dai X, Yang W, Wang Y, Woodgate R. Posttranslational regulation of human DNA polymerase iota. J Biol Chem 2015; E-pub ahead of print.
- McIntyre J, Woodgate R. Regulation of translesion DNA synthesis: posttranslational modification of lysine residues in key proteins. DNA Repair 2015; 29:166-179.
Collaborators
- Susana Cerritelli, PhD, Program in Genomics of Differentiation, NICHD, Bethesda, MD
- Michael Cox, PhD, University of Wisconsin, Madison, WI
- Robert Crouch, PhD, Program in Genomics of Differentiation, NICHD, Bethesda, MD
- Myron F. Goodman, PhD, University of Southern California, Los Angeles, CA
- Andrew Robinson, PhD, Rijksuniversiteit Groningen, Groningen, Netherlands
- Anton Simeonov, PhD, Scientific Director, NCATS, Bethesda, MD
- Antoine Van Oijen, PhD, University of Wollongong, Wollongong, Australia
- Digby Warner, PhD, University of Cape Town, Cape Town, South Africa
- Yinsheng Wang, PhD, University of California, Riverside, CA
- Wei Yang, PhD, Laboratory of Molecular Biology, NIDDK, Bethesda, MD
Contact
For more information, email woodgate@.nih.gov or visit www.nichd.nih.gov/research/atNICHD/Investigators/woodgate.