Global Regulation of Gene Expression by ppGpp
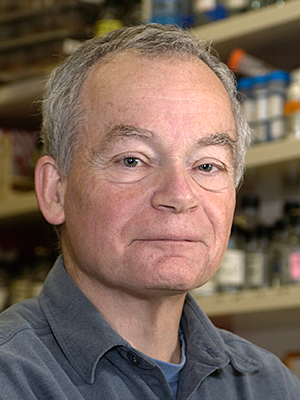
- Michael Cashel, MD, PhD, Head, Section on Molecular Regulation
- Llorenc Fernandez-Coll, PhD, Postdoctoral Intramural Research Training Award Fellow
- Nathan Thomas, MS, Postbaccalaureate Intramural Research Training Award Fellow
- Suanam Vij, AB, Postbaccalaureate Intramural Research Training Award Fellow
Our laboratory identified the structure and central regulatory features in ppGpp and pppGpp over 45 years ago, and we continue studies on these two nucleotide regulators of gene expression, which are analogs of GDP and GTP with a pyrophosphorylated ribose 3′-hydroxyl. The chemically unusual nucleotides are biologically ubiquitous and act as sensors of nutritional and environmental stress for bacteria, archea, plants, and arguably in animal cells. Sometimes called alarmones, they sense a bewildering variety of subtle changes in central metabolism and respond by triggering adaptive responses. Only two sensing mechanisms are well characterized; more are known but remain mechanistically elusive. The first mechanism that activates ppGpp synthetase senses even mild limitations of any charged tRNA species to keep up with the demands of protein synthesis, which serves to survey the metabolic availability of each amino acid, whether limited by de novo synthesis, by uptake, or by catabolism. The second known mechanism alters a conformational switch of a bifunctional hydrolase-synthetase enzyme when acylated or when nonacyl carrier protein (ACP) binds to the protein. The switch is thrown when lipids are limiting, which lowers rates of (p)ppGpp hydrolysis and activates (p)ppGpp synthesis leading to net accumulation. The circuit is thought to provide a broad sensor for changes in lipid, membrane physiology, energy metabolism, and possibly carbohydrate metabolism given that lipids are formed from assembly of two carbon units. Generally (p)ppGpp provokes very rapid physiological adjustments that circumvent the consequences of stress. This short-term adaptive cascade ultimately activates systems that ensure long-term survival.
Regulation by (p)ppGpp is global.
(p)ppGpp can alter about one third of total genomic expression at the level of transcription by interacting directly with RNA polymerase in Gram-negative bacteria. Gram-positive bacteria also regulate transcription with ppGpp, but indirectly by limiting GTP availability. Specific effects are noted as well with enzymes of protein synthesis, DNA synthesis, and intermediary metabolism. Past research from many laboratories, including our own, has focused on binding of ppGpp and pppGpp probably to two sites on RNA polymerase and how binding leads to promoter-specific regulation. The regulatory mechanism is complex and aided by a protein called DksA, which acts in concert with ppGpp to modify the kinetics of promoter initiation rather than classical inducer/repressor DNA sequence recognition. Different patterns of global regulation by ppGpp have emerged in different bacteria, which share abilities to exploit ppGpp to meet various stresses. A generic model is that, during stress, (p)ppGpp inhibits processes associated with luxuriant growth, which become superfluous during starvation, and activates functions that overcome the stress.
Regulation by (p)ppGpp is important.
The research has considerable therapeutic potential and has gained recently in popularity. (p)ppGpp has emerged as necessary for almost all pathogens to survive sources of stress imposed by hosts during infection. Several studies have documented key roles played by (p)ppGpp for enhancing virulence and required for a viable 'persistence' or tolerance of antibiotic-sensitive pathogens to the presence of antibiotics that target active metabolism. The most epidemiologically significant among bacterial pathogens is M. tuberculosis, whose persistence in carriers is globally epidemiologically significant. Key roles for ppGpp have also become evident for pathogens whose toxins are co-induced with bacteriophage lysogens, such as diphtheria and shigellosis. These features have led to ongoing searches for antibiotics aimed at limiting ppGpp synthesis. In addition, genetic manipulation of ppGpp has provided methods for dissecting out stages of pathogenesis, as defined by their (p)ppGpp dependence.
Our work is enlarging its focus.
We are collaborating to develop systematic approaches to discover new members of the (p)nNpp nucleotide family. Proof of principle of this approach is outlined in a book chapter (Potrykus and Cashel, Stress and Environmental Control of Gene Expression in Bacteria, Wiley, 2015;in press). The first example discovered is pGpp, the 5′ GMP derivative (Reference 3). This year, we concluded that biological approaches appear superior to custom structural design efforts to alter substrate specificities for ribosyl-3′ pyrophosphate hydrolysis. A network of functional interactions deduced from synthetic lethal phenotypic behavior was reported last year to include ppGpp, chaperones, and the omega RNA polymerase subunit during normal unstressed growth. This network of interactors during normal growth has now been extended to include the master chaperone GroEsEl, as well as involvement of gene functions that counter oxidative stress.
Characterization of a new regulator, pGpp
The early literature provides fleeting observations of unusual (p)ppGpp-like nucleotides, such as pGpp, ppGp, ppApp, pppAp and A5′p(pn)p5′Gpp, found in extracts of diverse bacteria when subjected to various sources of stress. We reported (Reference 3) that purified RelQ protein from the firmicute Enterococcus faecalis (RelQEF) catalyzes pyrophosphate transfer from ATP to the ribosyl-3′ hydroxyl of GMP without the need for additional supplements typically shown by RSH enzymes (ribosomes with an empty A site, mRNA, and codon-specified uncharged tRNA). The observation with RelQEF argues against the possibility that pGpp is a degradation product of (p)ppGpp formed by a nudix-like (phosphohydrolase) enzyme. It is notable that the metabolic turnover of pGpp, ppGpp, or pppGpp has not been reported in firmicutes, so there is a formal possibility that pGpp accumulation is regulated at the level of turnover. The kinetic rate constants for pGpp synthesis reveal RelQEF enzymatic efficiencies (Kcat/Km) within a factor of two of those for forming ppGpp or pppGpp, yet far below activities typical for RelEF, the RSH protein of E. faecalis. Both chemical and enzymatic labilities of pure pGpp (by RelEF) are similar to those of (p)ppGpp. We compared regulatory activities of pGpp with those known for ppGpp and pppGpp including PRPP–dependent 6-hydroxypurine transport, GMP kinase, transcription initiation of rRNA chains from an E. coli promoter, and regulatory effects on (p)ppGpp synthetases. Generally, we found pGpp regulatory activity to be quantitatively but not qualitatively different from that of ppGpp and pppGpp. A central question remains as to whether pGpp has unique regulatory functions that would serve to explain the evolutionary conservation of the enzyme.
Rational approach to understanding the structural basis of (p)ppNpp substrate specificity differences
Last year, we used a synthetic biology approach to obtain enzymes with dramatically different ribosyl-3′pyrophospho-hydrolase specific activities as an aid to find novel nucleotides. We took a designer approach because protein structures are available for two hydrolases, one G-specific and the other active towards A- and G-nucleotides. While in our laboratory, Katarzyna Potrykus verified the observations that the Mesh hydrolase is able to hydrolyze (p)ppGpp and discovered it could also hydrolyze (p)ppApp. Salient structural differences were modeled by Tamara James, while in our laboratory, and predictions were made for changes that might restrict the ability of Mesh to hydrolyze either (p)ppApp or (p)ppGpp. A set of nine mutant proteins were expressed, purified, their catalytic properties assessed, and their effects on catalytic properties used to generate three more mutants. Kinetic constants for hydrolysis were measured by a new assay developed in our laboratory and performed by Nathan Thomas. He found several mutants that lowered the efficiency of catalysis (kcat/km) relative to parental enzyme, but the effects applied equally for both substrates. Only two mutants were found to have a substrate preference: a five-fold higher efficiency for (p)ppApp for one and a three-fold preference for (p)ppGpp in the other. The changes may be useful for future modeling but are not strong enough for planned genetic approaches. Katarzyna Potrykus, now our collaborator in Gdansk, Poland, is pursuing these studies, exploring naturally occurring bacterial enzymes. The lack of specificity of the Mesh hydrolase may be sufficient for our purposes. Last year, we reported that expression of (p)ppA/Gpp Mesh hydrolase is toxic for cells unless (p)ppGpp is elevated. Given that (p)ppGpp is not essential for growth, an unknown Mesh substrate appears to be required for growth and may be spared by excess (p)ppGpp titrating out its hydrolysis. This behavior suggests that it is the hydrolase activity itself that is toxic rather than there being nonspecific effects of a toxic protein. Catalytically inactive Mesh proteins encountered among some members of our set of catalytic inactive missense mutants provide appropriate controls to rule out growth inhibition resulting from general protein toxicity.
Functional interaction network between ppGpp regulation, chaperones, the omega subunit of RNA polymerase and oxidative stress during rapid growth in rich media
Last year, we described synthetic lethal phenotypes for different combinations of deletion alleles: (1) the ppGpp synthetase gene (relA); (2) chaperones (dnaK, dnaJ, tig, clpB), and rpoZ, the RNA polymerase omega subunit. Functional interactions can be deduced if the phenotypes for combinations are more severe than the simple sum of the same deletions present singly. In this manner, it is observed that relA+, dnaK, or dnaJ enhance temperature sensitivity, i.e., show conditional synthetic lethality because one deletion functionally enhances the deficiency of the other. This is a plausible relation given that dnaK phenotypes are known to be suppressed by DksA, a known partner for ppGpp regulation. Similar interactions can be observed for triple deletions (relA and omega with either tig or clpB) or (relA, omega and groEsEl), further strengthening the links between ppGpp, chaperones, and the omega portion of the ppGpp–binding site of RNA polymerase. The work has been extended to include complementation assays, showing that transcription factor proteins (GreA and GreB) are able to complement the temperature sensitivity of a dnaK deletion. This is significant because GreA and GreB have superimposable protein structure with the DksA protein mentioned earlier, which acts both for ppGpp regulation and complementation of dnaK. Importantly, missense mutants of GreA, GreB, or DksA abolish their classical activities in transcription but do not abolish their ability to complement the defective chaperone phenotype of dnaK. This suggests that transcription factors have chaperone activities as 'moonlight' functions. The dksA mutant also complements the synthetic lethal triple mutant (relA, omega and tig), which appears to close the loop to include the three major channel factors, which are probably in the same functional network.
We made preliminary discoveries from gene libraries that complement relA-dnaK or relA-dnaJ synthetic lethality and therefore warrant further exploration. The functions of complementing genes predictably include those known for ppGpp regulation, chaperone, and transcription activity. Surprisingly, other complementing genes were found that mediate responses to oxidative stress, such as oxyR and catalases. We discovered over a decade ago that ppGpp regulates an alternative sigma factor (RpoS), which then activates catalases. The interconnections of these functions can be viewed as physiologically plausible, given that ppGpp functions to preclude damage from stress by adjustments to circumvent stress, while chaperone activities renature proteins denatured by stress conditions. However, the observations of synthetic lethality were made with cells growing luxuriantly in rich LB media under no apparent stress; nevertheless these functions appear to prevail as an operative functional stress network. This behavior suggests that there is a balance between these activities during normal growth, a balance that may even be necessary for normal growth. A few years ago, we published evidence that ppGpp is necessary and sufficient to control growth rate in rich as well as poor minimal media. Classically, this feature is usually interpreted in terms of ppGpp regulation of ribosome synthesis. Our current work suggests that other factors may be involved and opens approaches for discovering their identity.
Publications
- Mechold U, Potrykus K, Murphy H, Murakami KS, Cashel M. Differential regulation by ppGpp versus pppGpp in Escherichia coli. Nucleic Acids Res 2013; 41:6175-6189.
- Vinella D, Potrykus K, Murphy H, Cashel M. Effects on growth by changes of the balance between GreA, GreB and DksA suggest mutual competition and functional redundancy in Escherichia coli. J Bacteriol 2012; 194:261-273.
- Gaca AO, Kudrin P, Colomer-Winter C, Beljantseva J, Liu K, Anderson B, Wang JD, Rejman D, Potrykus K, Cashel M, Hauryliuk V, Lemos JA. From (p)ppGpp to (pp)pGpp: characterization of regulatory effects of pGpp synthesized by the small alarmone synthetase of Enterococcus faecalis. J Bacteriol 2015; 197(18):2908-2919.
Collaborators
- Jose Lemos, PhD, University of Rochester Medical Center School of Medicine and Dentistry, Rochester, NY
- Katarzyna Potrykus, PhD, University of Gdansk, Gdansk, Poland
- Evgeny Nudler, PhD, New York University School of Medicine, New York, NY
- Agnieszka Szalewska-Palasz, PhD, University of Gdansk, Gdansk, Poland
Contact
For more information, email cashel@mail.nih.gov or visit http://smr.nichd.nih.gov.