Cell Biophysics
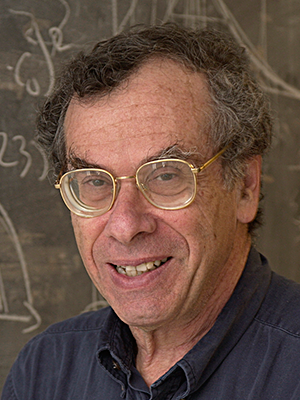
- Ralph Nossal, PhD, Head, Section on Cell Biophysics
- Dan Sackett, PhD, Staff Scientist
- Anand Banerjee, PhD, Visiting Fellow
- Felipe Montecinos, PhD, Visiting Fellow
- Alex Szatmary, PhD, Postdoctoral Fellow
- Hacène Boukari, PhD, Guest Researcher
- Norman Gershfeld, PhD, Guest Researcher
- Andrew Kalenkiewicz, BS, MSc, Postbaccalaureate Fellow
Our investigations focus on how cell behavior is linked to underlying physical mechanisms, for which we develop and apply methodologies based on mathematical and physical principles. Projects currently include: (1) elaborating a physical model to understand the dependence on mesoscopic mechanical parameters of eukaryotic cell uptake of viruses and nanoscopic drug-delivery particles via receptor-mediated endocytosis; 2) understanding the nature and consequences of temperature-linked phase transitions in biological cell membranes, particularly those occurring near the temperature at which the cells grow and are maintained; (3) optimizing the use of in vitro assays for amoeboid cell chemotaxis by assessing how such tests depend on cell sensitivity to chemotaxins, motility parameters of the putative chemotactic cells, and assay geometry; and (4) understanding how certain small molecules interact with microtubules and thereby act as antimitotic agents, and how microtubule arrays function in mitosis to produce accurate segregation of chromosomes. We have a particular interest in the ways cellular activities are coordinated in space and time.
Complex systems biophysics
For the past several years, we have been developing physical models to better understand the biogenesis of coated vesicles involved in clathrin-mediated endocytosis (CME) and other intracellular transport processes. CME is the principal pathway for the regulation of receptors and internalization of certain nutrients and signaling molecules at the plasma membrane of eukaryotic cells. Defects in CME can lead to metabolic disorders, aberrant signaling related to various cancers, and neurological disorders. A second major research area involves using published neurophysiological data to develop a deeper basic understanding of the biophysical behavior of cell membranes at the normal (growth or gestation) temperatures of organisms (see below). Additionally, in the recent past we have collaborated on the development of a mathematical model to explore how the difference in the average number of parasites (merozoites) released from malaria-infected blood cells can affect outcomes of therapy.
The early stage of receptor-mediated endocytosis involves the formation of transient structures known as clathrin-coated pits (CCPs). The process depends on the detailed energetics of protein binding and associated membrane transformations. The CCPs either mature into clathrin-coated vesicles (CCVs) or regress and vanish from the cell surface. During CCP formation, clathrin and several other proteins assemble to form a coat on the cytoplasmic side of the outer cell membrane. We previously developed a simple physical model for CCP dynamics and carried out Monte Carlo simulations to investigate the time development of CCP growth. Recently, we extended that model to investigate the role CME plays in the uptake of viruses and nanoparticles (NPs). Understanding how nano-sized particles interact with cell receptors and the clathrin coat is important when designing NPs for biomedical purposes as well as for developing strategies to inhibit cellular entry of viruses. Heretofore, theoretical models on this subject did not take clathrin coat assembly into explicit consideration. Thus, we developed a framework to study the endocytosis of single NPs that focuses on protein coat assembly and derived a simple analytical formula for the mean internalization time of NPs, defined as the average time between the binding of a NP to the plasma membrane and its entry into the cell. We studied the dependence of this quantity on coat parameters as well as NP size. There appears to be an optimal size at which cellular uptake is highest. Various published results indicate that the sizes appear to be independent of the type of cell, nanoparticle, or ligand. Moreover, experiments indicate that there is a maximum size beyond which uptake via clathrin-mediated endocytosis does not occur. We were able to show that such observations are consequences of the energetics and kinetics of protein coat formation during CCP production and predict how the efficacy of clathrin-mediated endocytosis depends on the mechanical properties of coat and membrane components (Reference 1 and manuscript in preparation).
We also investigated putative temperature-dependent lipid phase transitions occurring in higher eukaryotes. It is well established that microorganisms adjust the lipid composition of their membranes in response to changes in the temperature at which they are grown. Moreover, investigations carried out over many years demonstrated that whole lipid extracts from various higher organisms, as well as from microorganisms, exhibit singular properties at their growth temperatures. We investigated previously published data concerning the temperature dependence of the electrophysiological responses of cells obtained from representative animals (e.g., frog, squid, rat), searching for unusual features occurring at the gestation/growth temperature, Tg, of these animals. Special emphasis was given to the giant axons of the temperate squids Loligo forbesi and Loligo pealei, for which the temperature dependencies of the resting potentials and action potentials exhibit behaviors that strongly suggest the onset of a membrane state change at Tg, mirroring, in the case of squids, anomalies seen in the physical properties of films formed of whole lipid extracts obtained from those organisms. Based on approximations to the classic Hodgkin-Huxley equations, analysis of axonal responses indicates that observed changes in these electrophysiological characteristics most likely reflect reversible molecular couplings between voltage-switchable ion channels and surrounding lipids in the plasma membrane, which can affect the probability of channel opening. The change in electrophysiological properties with increasing temperature for these animals yields activation energies close to values noted for other putative lipid bilayer–linked kinetic processes that we have examined, as well as being seen in the diffusion of exogenous probes in lipid extracts (e.g., Jinet al., Biochemistry 1999;38:13275). These and other observations substantiate the view that, to first order, the lipid bilayer acts as a universal solvent for the embedded, integral proteins found in the plasma membrane.
Tubulin polymers and cytoskeletal organization
We continued our study of the properties of microtubules (MT), of drugs that alter these properties, and of the biology of MT arrays such as the mitotic spindle. In the course of this work, it was necessary to revisit the biophysical methods used for monitoring MT–targeting drug effects on cells exposed to the drugs. In addition, we addressed the mechanism that might underlie the potency of the drugs in treating patient tumors. We had previously presented evidence that this mechanism is not based on inhibition of mitosis, as often thought.
Microtubule-targeting drugs, and other chemotherapy agents, cause an increase in cellular reactive oxygen species (ROS) as a direct or ancillary effect of their intracellular action. Some such increases are known to be the result of effects on mitochondria, while others are attributable to other, often unknown, mechanisms. We pursued oxidative damage in cells by developing a fluorescent “tag” molecule that binds to and ‘lights up’ sites of oxidative damage inside cells, in particular carbonyl residues. We built on our previous use of “click-chemistry” to design a cell-permeable fluorophore that reacts with oxidized molecules. The molecules often contain carbonyl groups as a result of oxidation, and our click chemistry approach pairs a fluorophore with a hydrazine moiety to react spontaneously and specifically with the carbonyl groups. The result is the covalent attachment of a fluorophore to the sites of oxidation in the cell, which can then be visualized in the fluorescence microscope or identified biochemically following cell lysis and chromatography. We have now produced several variations on this oxidation sensor, with different fluorescence properties. We are currently attempting to make this approach work using fluorophores with longer excitation wavelengths than the current UV– or near UV–requiring molecule (Reference 2).
We also developed new fluorescent probe molecules that, in fluorescence microscopy, allow significant improvements in resolution. The probes utilize two wavelengths of light in a structured excitation beam to illuminate a sample and produce an image that has spatial resolution that is smaller than the limit set by the diffraction of light. The improved spatial resolution is achieved with this dye because it controls quenching of fluorescence emission by a “doughnut” of one wavelength of light, which effectively narrows the central, unquenched region to produce fluorescence emission due to the second wavelength of light from a spot smaller than the diffraction limit. The procedure is called Super-resolution via Transiently Activated Quencher, or STAQ. An advantage of the method, in addition to the improvement in spatial resolution, is the fact that the light power required for the procedure is much lower than the level required for other super-resolution methods. We are continuing to improve the dye and its applications (Reference 3).
MT–targeting drugs induce arrest of mitosis in rapidly dividing cells but also alter MT function during the 95 percent or more of the time that a cell is not in mitosis. The latter functions are likely to be the reason that such drugs are effective chemotherapy agents against many human cancers, given that mitosis is rare in tumors; and given that mitosis is not the main target of these drugs in tumors and that the drugs are effective against tumors, the target must be something else and present throughout the cell cycle, not only during mitosis. A candidate for the target is intracellular signaling and trafficking, which rely on microtubules to provide directional specificity. This may also underlie the well documented increase in clinical effectiveness observed when these agents are combined with DNA–damaging drugs. We hypothesized that the increased effectiveness occurs because the cells normally respond to DNA damage by recruiting DNA–repair enzymes from the cytoplasm to the nucleus. Such movement relies on microtubules to direct the traffic of the enzymes to the nucleus. Therefore, damaging the MT array with MT–targeting drugs blunts the cells’ ability to repair DNA damage, resulting in increased cell death. We tested the hypothesis with several cell lines and several combinations of DNA–damaging agents and MT–targeting agents, and the results appear to confirm our hypothesis. We are now extending our results to trafficking of other cancer-related proteins, such as oncogene proteins (Reference 4).
Biophysical methods and models
Our goal is to develop new methodologies to characterize the physical attributes and responsiveness of biological materials. Our work typically involves such techniques as mathematical modeling and simulation, polymer gel technology, and Fourier transform analysis of fluctuating optical signals. The work is directed towards a deeper understanding of the physical basis of quantitative measurement techniques and expanding the mathematical theory underlying related data analysis.
Thus we continued a project to provide the basis for quantitative assessment of the chemotactic response of neutrophils and other amoeboid cells. Migration of cells along gradients of effector molecules is necessary during an immune response and is involved in tissue development and cancer metastasis. The experimental assessment of chemotaxis is thus of great interest but is difficult to measure. Chemotaxis is frequently inferred by determining how many cells cross a boundary in a chemotaxis assay, for example how many cells crawl into the filter in the filter-migration assay or how many cells crawl into a defined region in the under agarose assay or agarose spot assay. The major limitation of these approaches is that such motion is not necessarily directed by the chemo-attractant gradient. To determine how reliably methods based on boundary crossing can indicate chemotactic motion of cells, we used information about the gradient sensitivity of neutrophils and MDA-MB-231 breast cancer cells to model how much gradient sensing increases the rate of boundary crossing relative to a random motility control, and over what duration. As part of this effort, we showed that neutrophils can sense chemo-attractant gradients generated in the under agarose and agarose spot assays for 1 to 2 hours. We also determined the chemo-attractant profile in the filter migration assay for filters of low porosity, and inferred that, in the filter assay, a neutrophil would be able to reliably perceive a gradient for about 10 hours. In contrast, chemotaxis of MDA-MB-231 cells, and cells with similar sensitivity to gradients, cannot be reliably measured by counting the cells in the agarose spot and under agarose assays. Moreover, although measurement of chemotaxis of these cells using the filter assay can be accomplished, doing so requires stringent controls. (Reference 5, and manuscript in preparation.)
A further activity involved the development of a method to track and quantify the movements of HIV viruses through dense mucus. We analyzed images obtained by time-resolved fluorescence confocal microscopy (t-FCM) and examined the motion of fluorescently labeled, inactivated HIV viruses (about 100 nm) that were added to samples of crude, untreated cervical mucus. After delineating the pixel locations of the fluorescent peaks associated with the viruses, we tracked the center of mass of their centroids over consecutive frames. To assess the randomness of the motion of each virus, we calculated changes of its statistical mean-squared displacement (MSD). As compared with our previous report (Boukari et al., Biomacromolecules 2009;10:2482), we doubled the observation time (about 34 second), which provided additional insight into the overall behavior of the viruses. Half the tracked viruses appeared significantly constrained, with their MSDs being very weakly dependent on time. The others showed relative mobility with MSDs that are proportional to ta + v2t2 over a time range t, depicting a combination of anomalous diffusion (a about 0–0.4) and/or slow, flow-like behavior. The MSD data reveal plateaus attributable to possible stalling and caging of the viruses during their motion, providing quantitative information that can guide the development of physical theories to deal with the way the heterogeneity and internal stresses of the mucus affect the movement of embedded nanoparticles.
Publications
- Banerjee A, Berezhkovskii A, Nossal R. Efficiency of cellular uptake of nanoparticles via receptor-mediated endocytosis. arXiv 2014; 14117348.
- Mukherjee K, Chio TI, Sackett DL, Bane SL. Detection of oxidative stress-induced carbonylation in live mammalian cells. Free Radic Biol Med 2015; 84:11-21.
- Rosales T, Sackett DL, Xu J, Shi ZD, Xu B, Li H, Kaur G, Frohart E, Shenoy N, Cheal SM, Wu H, Dulcey AE, Hu Y, Li C, Lane K, Griffiths GL, Knutson JR. STAQ: a route toward low power, multicolor nanoscopy. Microsc Res Tech 2015; 78:343-355.
- Poruchynsky MS, Komlodi-Pasztor E, Trostel S, Wilkerson J, Regairaz M, Pommier Y, Zhang X, Kumar Maity T, Robey R, Burotto M, Sackett D, Guha U, Fojo AT. Microtubule-targeting agents augment the toxicity of DNA-damaging agents by disrupting intracellular trafficking of DNA repair proteins. Proc Natl Acad Sci USA 2015; 112:1571-1576.
- Szatmary AC, Stuelten CH, Nossal R. Improving the design of the agarose spot assay for eukaryotic cell chemotaxis. RSC Adv 2014; 4:57343-57349.
Collaborators
- Susan Bane, PhD, Binghamton University, Binghamton, NY
- Alexander Berezhkovskii, PhD, Division of Computational Bioscience, CIT, NIH, Bethesda, MD
- Hacène Boukari, PhD, Delaware State University, Dover, DE
- Tito Fojo, MD, PhD, Medical Oncology Branch, NCI, Bethesda, MD
- Amir Gandjbakhche, PhD, Program in Pediatric Imaging and Tissue Sciences, NICHD, Bethesda, MD
- Svetlana Glushakova, MD, PhD, Program in Physical Biology, NICHD, Bethesda, MD
- Ferenc Horkay, PhD, Program in Pediatric Imaging and Tissue Sciences, NICHD, Bethesda, MD
- Albert J. Jin, PhD, Laboratory of Cellular Imaging and Macromolecular Biophysics, NIBIB, Bethesda, MD
- Jay Knutson, PhD, Laboratory of Molecular Biophysics, NHLBI, Bethesda, MD
- Eileen Lafer, PhD, University of Texas Health Science Center, San Antonio, TX
- Vladimir Larionov, PhD, Developmental Therapeutics Branch, NCI, Bethesda, MD
- Philip McQueen, PhD, Division of Computational Bioscience, CIT, NIH, Bethesda, MD
- Muraggapan Muthukumar, PhD, University of Massachusetts, Amherst, MA
- Carole Parent, PhD, Laboratory of Cellular and Molecular Biology, NCI, Bethesda, MD
- Thomas Ried, MD, Genetics Branch, Center for Cancer Research, NCI, Bethesda, MD
- Jennifer Ross, PhD, University of Massachusetts, Amherst, MA
- David Sept, PhD, University of Michigan, Ann Arbor, MI
- Christina Stuelten, MD, PhD, Laboratory of Cellular and Molecular Biology, Center for Cancer Research, NCI, Bethesda, MD
- Richard Taylor, PhD, Notre Dame University, Notre Dame, IN
- Al Yergey, PhD, Mass Spectrometry Core Facility, NICHD, Bethesda, MD
- Joshua Zimmerberg, MD, PhD, Program in Physical Biology, NICHD, Bethesda, MD
Contact
For more information, email nossalr@mail.nih.gov.